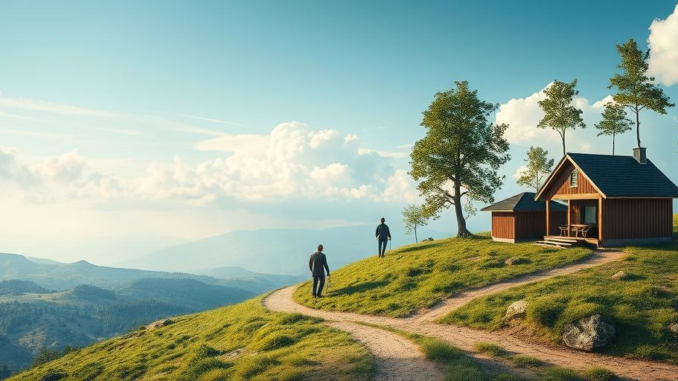
Beyond Cradle-to-Grave: A Critical Review of Lifecycle Assessment and Circular Economy Principles in the Built Environment
Abstract
Lifecycle Assessment (LCA) has emerged as a cornerstone methodology for evaluating the environmental burdens associated with building materials and construction processes. While traditionally focused on a ‘cradle-to-grave’ approach, encompassing extraction, manufacturing, use, and disposal, the growing imperative for sustainability necessitates a broader perspective. This research report critically examines the limitations of conventional LCA in the built environment and advocates for the integration of circular economy principles to achieve a truly holistic assessment of environmental impacts. The report explores the challenges of data scarcity and uncertainty in LCA, investigates strategies for promoting material durability and adaptability, delves into innovative waste management and circularity approaches, and evaluates the role of emerging technologies in facilitating lifecycle management. Furthermore, it emphasizes the importance of considering social and economic dimensions alongside environmental factors in the pursuit of sustainable construction practices. This review concludes that a paradigm shift towards ‘cradle-to-cradle’ thinking, supported by robust data infrastructure and policy frameworks, is crucial for minimizing the environmental footprint of the built environment and fostering a more sustainable future.
1. Introduction: The Evolving Landscape of Sustainable Construction
The construction industry is a significant contributor to global environmental impacts, accounting for a substantial portion of resource consumption, greenhouse gas emissions, and waste generation (UNEP, 2020). The urgency to mitigate these impacts has spurred the development and adoption of various sustainable construction practices, with Lifecycle Assessment (LCA) playing a pivotal role in quantifying and managing environmental performance.
Traditionally, LCA in the built environment has focused on a linear ‘cradle-to-grave’ model, encompassing the entire lifecycle of building materials and components, from raw material extraction and manufacturing to transportation, installation, use, maintenance, and eventual disposal (ISO 14040:2006). This approach provides valuable insights into the environmental hotspots within a product’s lifecycle, enabling informed decision-making regarding material selection, design optimization, and process improvements. However, the ‘cradle-to-grave’ approach has inherent limitations in addressing the complexities of long-lived assets like buildings, and the dynamic nature of material flows. Firstly, its end-of-life perspective often overlooks the potential for material recovery and reuse. Secondly, it may fail to adequately account for the long-term environmental consequences of material degradation, embodied carbon release, and potential land contamination.
In response to these limitations, there is a growing recognition of the need to transition towards a circular economy (CE) model in the built environment. The CE aims to decouple economic growth from resource consumption by minimizing waste and maximizing the value of materials and products through strategies such as reuse, repair, remanufacturing, and recycling (Ellen MacArthur Foundation, 2015). Integrating CE principles into LCA methodologies offers the potential to provide a more comprehensive and accurate assessment of the environmental impacts associated with building materials and construction processes, while also promoting resource efficiency and reducing waste.
This research report aims to critically examine the application of LCA in the built environment, focusing on its limitations and the potential for integration with circular economy principles. It explores the challenges of data availability and uncertainty, investigates strategies for promoting material durability and adaptability, delves into innovative waste management and circularity approaches, and evaluates the role of emerging technologies in facilitating lifecycle management. Furthermore, it emphasizes the importance of considering social and economic dimensions alongside environmental factors in the pursuit of truly sustainable construction practices. This review aims to provide a comprehensive understanding of the current state of LCA in the built environment and to identify opportunities for improvement in order to achieve a more sustainable and resource-efficient future.
2. Challenges and Limitations of Traditional LCA in the Built Environment
While LCA is a valuable tool for assessing the environmental impacts of building materials and construction processes, its application in the built environment faces several challenges and limitations. These challenges can significantly affect the accuracy and reliability of LCA results and hinder the effective implementation of sustainable construction practices.
2.1 Data Scarcity and Uncertainty
A primary challenge is the lack of comprehensive and reliable data on the environmental impacts of building materials and construction processes. LCA relies on extensive datasets covering a wide range of environmental indicators, such as energy consumption, greenhouse gas emissions, water usage, and waste generation. However, data for many building materials, particularly those that are novel or locally sourced, are often scarce or unavailable (Pomponi & Lenzen, 2018). This data gap necessitates the use of generic or proxy data, which can introduce significant uncertainty into the LCA results.
Furthermore, the quality of available data can vary considerably. Data may be outdated, incomplete, or based on different methodologies, making it difficult to compare and interpret results across different studies. The lack of transparency in data collection and reporting also poses a challenge, as it can be difficult to assess the reliability and validity of the data.
2.2 System Boundary Definition
Defining the system boundary in LCA can be complex, particularly for buildings with long lifespans and complex material compositions. The system boundary determines which processes and activities are included in the analysis, and it can significantly influence the results. Defining an appropriate system boundary requires careful consideration of the scope of the assessment, the availability of data, and the intended use of the results.
One common challenge is determining the appropriate timeframe for the LCA. Buildings typically have lifespans of 50 years or more, and the environmental impacts can vary significantly over time due to changes in energy sources, material degradation, and occupant behavior. Choosing an appropriate timeframe requires balancing the need for a comprehensive assessment with the limitations of data availability and the uncertainty of future events.
2.3 Allocation of Environmental Burdens
Allocation is another complex issue in LCA, particularly when dealing with multi-functional processes or products. Allocation refers to the process of assigning environmental burdens to different products or services that are produced jointly. For example, if a manufacturing process produces both a desired product and a waste byproduct, the environmental impacts of the process must be allocated between the two.
Several different allocation methods exist, including physical allocation, economic allocation, and system expansion. The choice of allocation method can significantly influence the LCA results, and there is no universally accepted method that is appropriate for all situations. This presents a challenge for LCA practitioners, who must carefully consider the specific context of the assessment and choose an allocation method that is justifiable and transparent.
2.4 Discounting and Long-Term Impacts
Long-term environmental impacts, such as climate change and resource depletion, pose a significant challenge for LCA. These impacts can extend far beyond the lifespan of a building, and their consequences may not be fully realized for decades or even centuries. Traditional LCA often uses discounting techniques to account for the time value of money, which can undervalue long-term environmental impacts. Discounting reduces the present value of future environmental costs, potentially leading to decisions that favor short-term economic benefits over long-term sustainability. The ethical implications of discounting and its impact on intergenerational equity are subject to ongoing debate.
2.5 Lack of Standardization and Harmonization
The lack of standardization and harmonization in LCA methodologies and data formats poses a significant challenge for comparing and interpreting results across different studies. Different LCA practitioners may use different methodologies, data sources, and system boundaries, making it difficult to compare their findings. This lack of comparability can hinder the effective implementation of sustainable construction practices and make it difficult to track progress towards sustainability goals. Efforts are underway to promote standardization and harmonization in LCA, but further progress is needed to ensure that LCA results are reliable, comparable, and transparent.
3. Integrating Circular Economy Principles into LCA
To overcome the limitations of traditional LCA and promote a more sustainable built environment, it is essential to integrate circular economy (CE) principles into LCA methodologies. This integration involves expanding the system boundary beyond ‘cradle-to-grave’ to include ‘cradle-to-cradle’ considerations, such as material recovery, reuse, remanufacturing, and recycling. By incorporating CE principles, LCA can provide a more comprehensive and accurate assessment of the environmental impacts associated with building materials and construction processes, while also promoting resource efficiency and reducing waste.
3.1 Extending the System Boundary: Cradle-to-Cradle Thinking
The ‘cradle-to-cradle’ approach recognizes that materials and products should be designed and managed in a way that allows them to be continuously cycled between different uses without losing their value or quality (McDonough & Braungart, 2002). This approach involves designing products for disassembly and reuse, using materials that are non-toxic and recyclable, and establishing closed-loop systems for material recovery and recycling. By extending the system boundary to include these ‘cradle-to-cradle’ considerations, LCA can capture the environmental benefits of material recovery and reuse and identify opportunities for closing material loops.
3.2 Modeling Material Recovery and Reuse
Integrating CE principles into LCA requires developing methods for modeling material recovery and reuse. This involves accounting for the energy and emissions associated with collecting, processing, and transporting materials for reuse or recycling. It also requires considering the quality and durability of recycled materials and their suitability for different applications.
One approach to modeling material recovery and reuse is to use a system expansion method, which involves comparing the environmental impacts of using recycled materials with the impacts of using virgin materials. This method can help to quantify the environmental benefits of recycling and identify opportunities for maximizing the use of recycled materials. However, it is important to account for the potential for ‘downcycling,’ where recycled materials are used in lower-value applications, which can reduce their environmental benefits.
3.3 Accounting for the Value of Material Stocks
Buildings represent significant material stocks that can be recovered and reused at the end of their useful life. However, traditional LCA often treats buildings as waste at the end-of-life stage, ignoring the potential for material recovery and reuse. To address this limitation, it is necessary to account for the value of material stocks in buildings and to develop strategies for maximizing their recovery and reuse. This involves conducting material flow analysis to identify the types and quantities of materials present in buildings and developing methods for assessing their potential for reuse or recycling.
3.4 Design for Disassembly and Adaptability
Design for disassembly (DfD) and design for adaptability (DfA) are crucial strategies for promoting circularity in the built environment. DfD involves designing buildings and components in a way that allows them to be easily disassembled at the end of their useful life, facilitating material recovery and reuse. DfA involves designing buildings to be flexible and adaptable to changing needs, extending their lifespan and reducing the need for new construction. By incorporating DfD and DfA principles into building design, it is possible to significantly reduce waste and promote the efficient use of resources over the entire lifecycle of a building. For example, modular construction systems and standardized components can facilitate disassembly and reuse, while flexible layouts and adaptable building systems can accommodate changing occupant needs and extend the lifespan of a building.
4. Strategies for Material Selection, Durability, and Adaptability
Selecting materials with lower environmental impacts, designing for durability, and promoting adaptability are essential strategies for reducing the environmental footprint of the built environment and fostering a circular economy. These strategies can significantly reduce resource consumption, minimize waste generation, and extend the lifespan of buildings.
4.1 Material Selection Criteria
When selecting materials for construction, it is important to consider a range of environmental factors, including embodied energy, greenhouse gas emissions, water usage, and toxicity. Materials with lower embodied energy and emissions are generally preferable, as they contribute less to climate change. Materials that are sourced locally and manufactured using sustainable practices are also desirable. Furthermore, materials should be non-toxic and safe for human health and the environment. Preference should be given to materials with Environmental Product Declarations (EPDs), which provide transparent and verified information about their environmental impacts. EPDs are based on LCA and provide standardized information about the environmental performance of a product across its entire lifecycle. Tools like the BREEAM and LEED rating systems award points for using materials with EPDs, encouraging manufacturers to be transparent about the environmental impact of their products.
4.2 Enhancing Durability and Longevity
Designing for durability is crucial for extending the lifespan of buildings and reducing the need for frequent repairs and replacements. Durable materials and construction techniques can significantly reduce resource consumption and waste generation over the long term. Factors such as climate, exposure to moisture, and occupant behavior should be considered when selecting materials and designing building systems. Materials should be resistant to degradation, corrosion, and other forms of damage. Proper detailing and construction practices are also essential for ensuring the durability of buildings. For example, using appropriate waterproofing systems and providing adequate ventilation can help to prevent moisture damage and extend the lifespan of building components.
4.3 Promoting Adaptability and Flexibility
Designing for adaptability is essential for ensuring that buildings can meet the changing needs of occupants over time. Adaptable buildings can be easily reconfigured or repurposed to accommodate different uses, extending their lifespan and reducing the need for new construction. This can be achieved through flexible layouts, modular construction systems, and adaptable building systems. Flexible layouts allow spaces to be easily reconfigured to accommodate different activities or occupancy patterns. Modular construction systems allow buildings to be easily expanded or reconfigured, while adaptable building systems allow for changes in heating, cooling, and lighting systems to meet changing energy needs. Designing for adaptability requires considering the potential future uses of a building and incorporating features that allow for easy modification and adaptation.
5. Waste Management and Circularity in Construction
The construction industry is a significant generator of waste, and effective waste management practices are essential for reducing the environmental impact of construction projects. Circularity in construction aims to minimize waste generation by promoting the reuse, recycling, and recovery of materials. This involves adopting innovative waste management techniques, implementing closed-loop material systems, and promoting collaboration among stakeholders.
5.1 Waste Minimization Strategies
Waste minimization strategies should be implemented at all stages of the construction process, from design to demolition. This includes designing for material efficiency, optimizing construction processes, and implementing effective waste segregation and recycling programs. Designing for material efficiency involves minimizing the amount of materials used in construction without compromising structural integrity or performance. This can be achieved through careful planning, detailed design, and the use of lightweight materials. Optimizing construction processes involves minimizing waste generation during construction activities through careful planning, efficient material handling, and proper training of workers. Effective waste segregation and recycling programs involve separating different types of waste on site and sending them to appropriate recycling facilities.
5.2 Deconstruction and Material Recovery
Deconstruction is the process of carefully dismantling a building in order to recover and reuse its materials. Deconstruction is a more sustainable alternative to demolition, as it allows for the recovery of valuable materials that would otherwise be landfilled. Deconstruction requires careful planning and skilled labor, but it can provide significant environmental and economic benefits. Materials recovered from deconstruction can be reused in new construction projects, reducing the demand for virgin materials and minimizing waste generation.
5.3 Closed-Loop Material Systems
Closed-loop material systems aim to create a circular flow of materials, where waste is minimized and materials are continuously recycled and reused. This involves establishing partnerships between manufacturers, contractors, and recyclers to ensure that materials are properly collected, processed, and reused. Closed-loop systems can be implemented for a variety of materials, including concrete, steel, wood, and plastics. For example, concrete can be crushed and recycled into aggregate for new concrete or road construction. Steel can be melted down and recycled into new steel products. Wood can be reused in new construction or processed into wood chips for landscaping or fuel.
6. Tools and Technologies for Lifecycle Management
Several tools and technologies are available to help track and manage the lifecycle impacts of building materials and components. These tools can assist in material selection, design optimization, and waste management, facilitating the implementation of sustainable construction practices.
6.1 Building Information Modeling (BIM)
Building Information Modeling (BIM) is a digital representation of a building that can be used to manage information throughout its entire lifecycle. BIM can be used to track the materials used in construction, assess their environmental impacts, and optimize building design for sustainability. BIM can also be used to manage waste during construction and demolition, facilitating material recovery and reuse. BIM allows for the creation of a virtual model of a building that contains detailed information about its components, materials, and systems. This information can be used to perform LCA, track material flows, and optimize building performance.
6.2 Lifecycle Assessment Software
Several software tools are available for performing LCA on building materials and construction processes. These tools can help to quantify the environmental impacts of different materials and design options, allowing for informed decision-making. LCA software typically includes databases of environmental data for a wide range of materials and processes, as well as tools for modeling material flows and calculating environmental impacts. Some popular LCA software tools include SimaPro, GaBi, and openLCA.
6.3 Material Tracking Systems
Material tracking systems can be used to track the flow of materials throughout the construction process, from manufacturing to installation to demolition. These systems can help to identify opportunities for waste reduction and material recovery, as well as to ensure that materials are properly handled and disposed of. Material tracking systems can be implemented using a variety of technologies, including barcodes, RFID tags, and GPS tracking. These systems can provide real-time information about the location and status of materials, allowing for better management and control.
6.4 Digital Platforms for Material Exchange
Digital platforms for material exchange can facilitate the reuse and recycling of building materials by connecting buyers and sellers of used materials. These platforms can help to create a market for recovered materials, reducing waste and promoting circularity. Digital platforms for material exchange can be used to list and sell a variety of materials, including lumber, bricks, concrete, and steel. These platforms can also provide information about the environmental benefits of using recovered materials. They are also sometimes referred to as material passports.
7. Social and Economic Considerations
While environmental performance is a primary focus of LCA and circular economy initiatives, it is crucial to consider social and economic dimensions to ensure truly sustainable construction practices. Integrating these factors ensures that sustainability efforts benefit both the environment and society as a whole.
7.1 Social Impact Assessment
Social Impact Assessment (SIA) should be integrated into the LCA framework to evaluate the social consequences of material choices and construction practices. This includes assessing the impact on worker health and safety, community well-being, and local economies. For instance, the extraction and processing of certain materials may involve hazardous working conditions or displacement of communities. SIA helps identify and mitigate these negative social impacts. Furthermore, promoting the use of locally sourced materials can support local businesses and create jobs within the community.
7.2 Economic Feasibility and Cost-Benefit Analysis
Economic feasibility is a critical factor in the adoption of sustainable construction practices. While some sustainable materials and technologies may have higher upfront costs, they can often provide long-term cost savings through reduced energy consumption, lower maintenance costs, and extended lifespan. Cost-benefit analysis (CBA) can be used to evaluate the economic viability of different sustainability initiatives, taking into account both the costs and benefits over the entire lifecycle of a building. It is also important to consider the external costs associated with environmental degradation and resource depletion, which are often not reflected in market prices. The increased popularity of green bonds and sustainable finance options is also driving down the cost of investing in sustainable construction.
7.3 Stakeholder Engagement and Collaboration
Effective stakeholder engagement is essential for the successful implementation of sustainable construction practices. This involves engaging with architects, engineers, contractors, material suppliers, building owners, and occupants to ensure that their needs and concerns are addressed. Collaboration among stakeholders can help to identify innovative solutions, overcome barriers, and promote the adoption of best practices. Furthermore, engaging with local communities can help to ensure that construction projects are aligned with their values and priorities. Public awareness campaigns and educational programs can also help to raise awareness about the benefits of sustainable construction and encourage widespread adoption.
8. Conclusion and Future Directions
This research report has critically examined the application of Lifecycle Assessment (LCA) in the built environment, highlighting its limitations and advocating for the integration of circular economy principles. While traditional LCA provides valuable insights into the environmental impacts of building materials and construction processes, it often fails to adequately address the complexities of long-lived assets and the potential for material recovery and reuse.
To overcome these limitations, a paradigm shift towards ‘cradle-to-cradle’ thinking is essential. This involves extending the system boundary of LCA to include material recovery, reuse, remanufacturing, and recycling, and developing methods for modeling these processes accurately. Furthermore, it requires promoting design for disassembly and adaptability, selecting materials with lower environmental impacts, and implementing effective waste management and circularity practices.
Future research should focus on developing more comprehensive and reliable datasets for LCA, improving methods for modeling material recovery and reuse, and exploring the social and economic dimensions of sustainable construction. Emerging technologies, such as Building Information Modeling (BIM) and material tracking systems, can play a crucial role in facilitating lifecycle management and promoting the adoption of sustainable construction practices.
The construction industry has a significant opportunity to reduce its environmental footprint and contribute to a more sustainable future. By embracing LCA and circular economy principles, the built environment can become a catalyst for innovation, resource efficiency, and social responsibility.
References
- Ellen MacArthur Foundation. (2015). Towards a circular economy: Business rationale for an accelerated transition. Ellen MacArthur Foundation.
- ISO 14040. (2006). Environmental management – Life cycle assessment – Principles and framework. International Organization for Standardization.
- McDonough, W., & Braungart, M. (2002). Cradle to cradle: Remaking the way we make things. North Point Press.
- Pomponi, F., & Lenzen, M. (2018). Environmental assessment of construction materials: A review. Journal of Cleaner Production, 172, 2221-2230.
- UNEP. (2020). Global Status Report for Buildings and Construction 2020. United Nations Environment Programme.
“Cradle-to-cradle,” eh? Sounds less like construction and more like advanced baby-proofing. Should we start stocking LEED-certified teething rings and eco-friendly playpens? Asking for a friend building a daycare… allegedly.
Haha, love the analogy! LEED-certified teething rings might be taking it a bit far, but the idea of creating healthier, safer spaces for our little ones is spot on. Using non-toxic materials and optimizing indoor air quality in daycares is definitely something we should prioritize! What materials would you consider first?
Editor: FocusNews.Uk
Thank you to our Sponsor Focus 360 Energy
“Cradle-to-cradle” sounds lovely… until you’re trying to explain to the demolition crew that, no, they can’t just smash it all to bits. We’re aiming for architectural reincarnation, people! Anyone got a handy guide to convince them that carefully dismantling a skyscraper is a better workout than wrecking ball demolition?
That’s a fantastic point! The practicalities of ‘architectural reincarnation’ definitely present challenges. Perhaps offering training in deconstruction techniques could be beneficial, highlighting the value of material recovery and the potential for creating new jobs in the circular economy. Does anyone know of successful deconstruction training programs?
Editor: FocusNews.Uk
Thank you to our Sponsor Focus 360 Energy