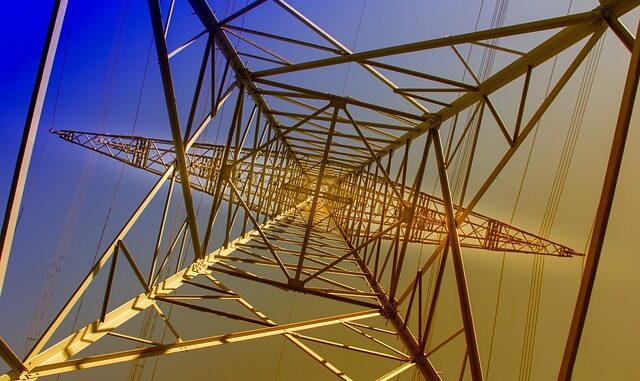
Abstract
The built environment, encompassing infrastructure, buildings, and urban landscapes, is undergoing a profound transformation driven by rapid technological advancements. This research report provides a comprehensive analysis of the pervasive impact of technology on the built environment, moving beyond specific software applications like project management tools or BIM to explore broader systemic changes. It examines the influence of digital technologies across various stages of the lifecycle of the built environment, from initial planning and design to construction, operation, and eventual decommissioning. Furthermore, the report critically evaluates the challenges and opportunities presented by these technologies, including issues related to data security, ethical considerations, workforce adaptation, and the potential for exacerbating existing inequalities. Finally, it explores emerging trends and future trajectories, considering the transformative potential of artificial intelligence, advanced robotics, digital twins, and sustainable materials in shaping the future of the built environment. This report aims to provide a holistic and nuanced understanding of the multifaceted relationship between technology and the built environment, offering insights for researchers, policymakers, and industry professionals.
Many thanks to our sponsor Focus 360 Energy who helped us prepare this research report.
1. Introduction: Technology as a Catalyst for Change
The built environment, traditionally characterized by its slow pace of innovation, is now experiencing a period of rapid transformation fueled by technological advancements. From the development of novel construction materials to the integration of smart building systems, technology is fundamentally reshaping how we design, construct, and interact with the physical spaces around us. This transformation is not merely about incremental improvements in existing processes; rather, it represents a paradigm shift towards a more efficient, sustainable, and human-centric built environment.
While specific technologies like Building Information Modeling (BIM) and project management software have garnered significant attention for their ability to streamline workflows and improve project outcomes, their true value lies in their capacity to facilitate broader systemic changes. BIM, for example, transcends its role as a 3D modeling tool to become a centralized repository of building information, enabling enhanced collaboration, clash detection, and lifecycle management (Eastman et al., 2018). Similarly, project management software not only improves scheduling and resource allocation but also provides valuable data insights that can inform future projects and optimize decision-making.
This research report adopts a broader perspective, examining the multifaceted impact of technology on the built environment. It explores the influence of digital technologies across various stages of the lifecycle, from initial planning and design to construction, operation, and eventual decommissioning. Moreover, the report critically evaluates the challenges and opportunities presented by these technologies, including issues related to data security, ethical considerations, workforce adaptation, and the potential for exacerbating existing inequalities. Finally, it explores emerging trends and future trajectories, considering the transformative potential of artificial intelligence, advanced robotics, digital twins, and sustainable materials in shaping the future of the built environment.
Many thanks to our sponsor Focus 360 Energy who helped us prepare this research report.
2. Digital Design and Planning: Reimagining the Creative Process
The initial stages of design and planning are being revolutionized by digital technologies that empower architects and engineers to create more complex, innovative, and sustainable solutions. Computer-Aided Design (CAD) software, initially introduced in the late 20th century, laid the groundwork for digital design, allowing for the creation of precise and detailed drawings. However, contemporary digital design tools extend far beyond simple drafting, incorporating advanced simulation, optimization, and visualization capabilities.
2.1 Generative Design: Generative design represents a significant leap forward, utilizing algorithms and artificial intelligence to automatically generate multiple design options based on specified constraints and performance criteria. This approach allows designers to explore a wider range of possibilities, identify optimal solutions, and optimize designs for specific goals, such as energy efficiency, structural performance, or cost-effectiveness. For example, Autodesk’s Dreamcatcher platform allows users to define design goals and constraints, and the software generates numerous design options, enabling designers to make informed decisions based on data-driven insights (Autodesk, 2023).
2.2 Parametric Modeling: Parametric modeling allows for the creation of intelligent 3D models where design parameters are interconnected, enabling rapid exploration of design variations and automated adjustments based on changing conditions. This approach is particularly valuable for complex geometries and repetitive design elements, allowing designers to quickly iterate and optimize designs for specific performance criteria (Kolarevic, 2003). Tools like Grasshopper, a visual programming language integrated with Rhinoceros 3D, enable designers to create custom parametric models and automate complex design workflows (Rutten, 2007).
2.3 Virtual and Augmented Reality (VR/AR): Virtual and augmented reality technologies offer immersive and interactive experiences for design review and stakeholder engagement. VR allows users to experience designs in a fully immersive virtual environment, providing a realistic sense of scale and spatial relationships. AR overlays digital information onto the real world, allowing designers to visualize how a building will interact with its surrounding environment. These technologies can improve communication, facilitate collaboration, and reduce errors by enabling stakeholders to experience designs before construction begins (Whyte, 2002).
The integration of these digital design tools is not without its challenges. The complexity of these technologies requires specialized training and expertise, and the transition to digital workflows can be disruptive for established firms. Moreover, the reliance on digital tools raises questions about the role of human creativity and intuition in the design process. It’s important to see these technologies as tools that augment, rather than replace, human design capabilities, allowing designers to focus on higher-level creative tasks and strategic decision-making.
Many thanks to our sponsor Focus 360 Energy who helped us prepare this research report.
3. Construction Technology: Optimizing Efficiency and Safety
The construction industry, historically slow to adopt new technologies, is now embracing a wide range of innovations that promise to improve efficiency, reduce costs, and enhance safety. From automated construction equipment to drone-based site monitoring, technology is transforming every aspect of the construction process.
3.1 Building Information Modeling (BIM): As mentioned earlier, BIM is far more than just a 3D modeling tool. It serves as a central repository of building information, integrating design, construction, and operational data into a single, comprehensive model. This enables enhanced collaboration, clash detection, and lifecycle management, leading to improved project outcomes and reduced errors. BIM’s value extends beyond the design and construction phases, facilitating facility management and maintenance throughout the building’s lifecycle (Eastman et al., 2018).
3.2 Robotics and Automation: Robotics and automation are playing an increasingly important role in construction, performing repetitive and physically demanding tasks with greater speed and precision. Examples include automated bricklaying robots, concrete printing systems, and robotic demolition equipment. These technologies can improve productivity, reduce labor costs, and enhance worker safety by minimizing exposure to hazardous conditions (Bock & Linner, 2015).
3.3 Drones and Site Monitoring: Drones equipped with cameras and sensors are being used to monitor construction sites, providing real-time aerial imagery and data that can be used to track progress, identify potential safety hazards, and optimize resource allocation. Drone-based surveys can also be used to create accurate 3D models of construction sites, facilitating site planning and coordination. These technologies provide valuable insights that can improve project management and reduce delays (Irizarry et al., 2013).
3.4 3D Printing (Additive Manufacturing): 3D printing, also known as additive manufacturing, is emerging as a potentially disruptive technology in construction, allowing for the creation of complex and customized building components directly from digital models. 3D-printed homes and building elements are becoming increasingly common, offering the potential for faster construction times, reduced material waste, and greater design flexibility. While challenges remain in terms of scalability and material performance, 3D printing holds significant promise for the future of construction (Lim et al., 2012).
However, the adoption of these construction technologies faces several challenges. The high initial investment costs, the need for specialized training, and the resistance to change within the industry can hinder their widespread adoption. Furthermore, concerns about job displacement due to automation need to be addressed through workforce training and reskilling programs. The integration of these technologies requires a holistic approach that considers the entire construction ecosystem, including supply chains, regulatory frameworks, and workforce capabilities.
Many thanks to our sponsor Focus 360 Energy who helped us prepare this research report.
4. Smart Buildings and the Internet of Things (IoT): Enhancing Occupant Experience and Operational Efficiency
Smart buildings, equipped with sensors, actuators, and communication networks, are transforming how buildings are operated and managed. The Internet of Things (IoT) enables the integration of various building systems, such as HVAC, lighting, security, and energy management, allowing for centralized control and optimization. This leads to improved occupant experience, reduced energy consumption, and enhanced operational efficiency.
4.1 Building Management Systems (BMS): BMS systems collect and analyze data from various building sensors, providing insights into building performance and enabling automated control of building systems. This allows for optimized energy consumption, improved indoor air quality, and enhanced security. Modern BMS systems are increasingly integrated with cloud-based platforms, enabling remote monitoring and control (Buckman et al., 2014).
4.2 Occupancy Sensors and Space Optimization: Occupancy sensors detect the presence of people in a space, allowing for automated adjustments to lighting, HVAC, and other building systems based on actual occupancy levels. This can significantly reduce energy waste and improve occupant comfort. Data from occupancy sensors can also be used to optimize space utilization, identifying underutilized areas and informing space planning decisions (Erickson & Cerpa, 2011).
4.3 Predictive Maintenance: IoT sensors can monitor the condition of building equipment, such as HVAC systems and elevators, providing early warnings of potential failures. This allows for predictive maintenance, enabling proactive repairs and preventing costly breakdowns. Predictive maintenance can significantly reduce downtime, extend the lifespan of building equipment, and improve overall operational efficiency (Jardine et al., 2006).
4.4 Smart Grids and Energy Management: Smart buildings can be integrated with smart grids, allowing for dynamic energy pricing and demand response programs. This enables buildings to reduce their energy consumption during peak demand periods and contribute to a more stable and sustainable energy grid. Smart building technologies can also facilitate the integration of renewable energy sources, such as solar panels and wind turbines, reducing the building’s carbon footprint (Hammerstrom, 2007).
The implementation of smart building technologies raises important questions about data privacy and security. The vast amount of data collected by building sensors needs to be protected from unauthorized access and misuse. Robust cybersecurity measures and clear data privacy policies are essential to ensure the responsible use of smart building technologies. Furthermore, the complexity of these systems requires specialized expertise for installation, maintenance, and operation. Training and education programs are needed to develop a skilled workforce capable of managing and optimizing smart building technologies.
Many thanks to our sponsor Focus 360 Energy who helped us prepare this research report.
5. Sustainable Materials and Construction Techniques: Building for a Greener Future
The built environment is a major contributor to greenhouse gas emissions and resource depletion. Sustainable materials and construction techniques are essential for mitigating the environmental impact of the built environment and creating a more sustainable future. Technology plays a crucial role in developing and implementing these solutions.
5.1 Life Cycle Assessment (LCA): Life Cycle Assessment (LCA) is a methodology for evaluating the environmental impacts of a product or building throughout its entire lifecycle, from raw material extraction to end-of-life disposal. LCA tools can be used to compare the environmental performance of different materials and construction techniques, informing decisions about material selection and design optimization. LCA can help identify opportunities to reduce environmental impacts at each stage of the lifecycle (ISO 14040, 2006).
5.2 Sustainable Building Materials: A wide range of sustainable building materials are available, including recycled materials, renewable materials, and materials with low embodied energy. Examples include recycled concrete aggregate, bamboo, wood certified by the Forest Stewardship Council (FSC), and bio-based materials like hempcrete. Technology is playing a role in developing new and innovative sustainable materials, such as self-healing concrete and carbon-sequestering materials (Ashby, 2012).
5.3 Prefabrication and Modular Construction: Prefabrication and modular construction involve manufacturing building components off-site in a controlled factory environment, reducing construction waste, improving quality control, and accelerating construction times. These techniques can also reduce the environmental impact of construction by minimizing site disturbance and optimizing material usage. Technology, such as BIM and automated manufacturing processes, is essential for designing and fabricating modular building components (Goodier & Gibb, 2003).
5.4 Green Building Standards and Certifications: Green building standards and certifications, such as LEED (Leadership in Energy and Environmental Design) and BREEAM (Building Research Establishment Environmental Assessment Method), provide frameworks for designing and constructing high-performance buildings. These standards encourage the use of sustainable materials, energy-efficient design, and water conservation measures. Technology plays a role in monitoring and verifying building performance to ensure compliance with these standards (USGBC, 2023; BRE, 2023).
However, the adoption of sustainable materials and construction techniques faces several challenges. The initial cost of sustainable materials can be higher than conventional materials, and the lack of awareness and knowledge about sustainable building practices can hinder their widespread adoption. Moreover, regulatory barriers and a lack of incentives can discourage developers from investing in sustainable building projects. Addressing these challenges requires a combination of policy interventions, market incentives, and education and training programs.
Many thanks to our sponsor Focus 360 Energy who helped us prepare this research report.
6. Challenges and Opportunities: Navigating the Future of Technology in the Built Environment
The integration of technology into the built environment presents both significant challenges and unprecedented opportunities. Addressing these challenges and leveraging these opportunities is crucial for realizing the full potential of technology in shaping a more efficient, sustainable, and human-centric built environment.
6.1 Data Security and Privacy: The increasing reliance on data in the built environment raises important questions about data security and privacy. Building sensors collect vast amounts of data about building occupants, their behavior, and building performance. This data needs to be protected from unauthorized access and misuse. Robust cybersecurity measures, clear data privacy policies, and ethical guidelines are essential for ensuring the responsible use of data in the built environment (Weber, 2015).
6.2 Workforce Adaptation and Reskilling: The adoption of new technologies in the built environment requires a skilled workforce capable of operating, maintaining, and optimizing these technologies. Workforce training and reskilling programs are needed to prepare workers for the changing demands of the industry. This includes training in areas such as BIM, robotics, data analytics, and sustainable building practices. It’s also important to address potential job displacement due to automation by creating new job opportunities in emerging fields (Manyika et al., 2017).
6.3 Ethical Considerations and Bias: Algorithms and artificial intelligence are increasingly used in the built environment, from generative design to predictive maintenance. It’s important to ensure that these algorithms are fair, unbiased, and transparent. Biased algorithms can perpetuate existing inequalities and discriminate against certain groups. Ethical guidelines and regulatory frameworks are needed to ensure the responsible development and deployment of AI in the built environment (O’Neil, 2016).
6.4 Cost and Accessibility: The initial cost of implementing new technologies can be a barrier to adoption, particularly for small and medium-sized enterprises (SMEs). Ensuring that these technologies are accessible and affordable is crucial for promoting widespread adoption and preventing the creation of a digital divide. Government subsidies, tax incentives, and open-source software initiatives can help reduce the cost of technology and improve accessibility.
6.5 Regulatory Frameworks and Standards: Regulatory frameworks and standards need to adapt to the rapid pace of technological change in the built environment. Outdated regulations can stifle innovation and prevent the adoption of new technologies. Governments need to work with industry stakeholders to develop flexible and adaptive regulatory frameworks that promote innovation while ensuring safety and sustainability (OECD, 2017).
Despite these challenges, the opportunities presented by technology in the built environment are immense. Technology can help us create more efficient, sustainable, and resilient buildings and infrastructure. It can improve the quality of life for building occupants, reduce environmental impacts, and drive economic growth. By addressing the challenges and leveraging the opportunities, we can harness the power of technology to shape a better future for the built environment.
Many thanks to our sponsor Focus 360 Energy who helped us prepare this research report.
7. Future Trajectories: Emerging Trends and Transformative Technologies
The built environment is poised for further transformation as emerging technologies continue to mature and converge. These technologies hold the potential to revolutionize how we design, construct, operate, and interact with the built environment.
7.1 Artificial Intelligence (AI) and Machine Learning (ML): AI and ML are already playing an increasingly important role in the built environment, and their impact is expected to grow significantly in the coming years. AI can be used for a wide range of applications, including generative design, predictive maintenance, energy optimization, and smart city planning. ML algorithms can analyze vast amounts of data to identify patterns and predict future outcomes, enabling more informed decision-making (Jordan & Mitchell, 2015).
7.2 Digital Twins: Digital twins are virtual representations of physical assets, such as buildings or infrastructure, that are updated in real-time with data from sensors and other sources. Digital twins can be used to monitor the performance of assets, identify potential problems, and optimize operations. They can also be used for simulation and testing, allowing engineers to explore different scenarios and optimize designs before construction begins (Glaessgen & Stargel, 2012).
7.3 Blockchain Technology: Blockchain technology offers a secure and transparent way to manage transactions and data in the built environment. Blockchain can be used for applications such as supply chain management, smart contracts, and land registry. It can improve transparency, reduce fraud, and streamline processes (Swan, 2015).
7.4 Advanced Materials: Research and development efforts are focused on creating new and innovative materials with enhanced performance characteristics, such as self-healing concrete, carbon-sequestering materials, and lightweight composites. These materials can improve the durability, sustainability, and resilience of buildings and infrastructure (Meyers & Chawla, 2009).
7.5 Bio-integrated Design: Bio-integrated design explores the integration of living organisms into the built environment, creating buildings and infrastructure that are more sustainable, resilient, and responsive to their environment. Examples include green roofs, living walls, and bio-solar panels. This approach has the potential to transform the built environment into a more integrated and symbiotic part of the natural world (Allen & Gilliam, 2018).
These emerging trends and transformative technologies are shaping the future of the built environment, creating new opportunities for innovation and collaboration. By embracing these technologies and addressing the associated challenges, we can create a built environment that is more efficient, sustainable, and human-centric.
Many thanks to our sponsor Focus 360 Energy who helped us prepare this research report.
8. Conclusion
Technology is fundamentally transforming the built environment, impacting every stage of the lifecycle from design and construction to operation and decommissioning. This research report has provided a comprehensive overview of the pervasive impact of technology, exploring the influence of digital technologies across various domains, including digital design, construction technology, smart buildings, sustainable materials, and emerging trends. While the adoption of these technologies presents several challenges related to data security, workforce adaptation, ethical considerations, and cost accessibility, the potential benefits are immense. These benefits include enhanced efficiency, improved sustainability, enhanced occupant experience, and increased resilience.
The future of the built environment will be shaped by the convergence of emerging technologies such as artificial intelligence, digital twins, blockchain, advanced materials, and bio-integrated design. These technologies offer unprecedented opportunities for innovation and collaboration, enabling the creation of a built environment that is more efficient, sustainable, human-centric, and integrated with the natural world. To realize the full potential of technology in the built environment, it is crucial to address the associated challenges through proactive policy interventions, workforce training programs, ethical guidelines, and collaborative partnerships. By embracing innovation and addressing the challenges, we can harness the transformative power of technology to create a better future for the built environment and the communities it serves.
Many thanks to our sponsor Focus 360 Energy who helped us prepare this research report.
References
- Allen, B. E., & Gilliam, F. S. (2018). The ecological engineer. Island Press.
- Ashby, M. F. (2012). Materials and the environment: Eco-informed material choice. Butterworth-Heinemann.
- Autodesk. (2023). Dreamcatcher. https://www.autodesk.com/solutions/generative-design/manufacturing
- Bock, T., & Linner, T. (2015). Mass customization in construction: Current state and future trends. John Wiley & Sons.
- BRE. (2023). BREEAM. https://www.breeam.com/
- Buckman, A. H., Mayfield, M., & Beck, S. B. (2014). Smart building management systems. ASHRAE Journal, 56(10), 36-45.
- Eastman, C., Teicholz, P., Sacks, R., & Liston, K. (2018). BIM handbook: A guide to building information modeling for owners, managers, designers, engineers, and contractors. John Wiley & Sons.
- Erickson, V. L., & Cerpa, N. (2011). Occupancy detection for demand-controlled building systems. IEEE Sensors Journal, 11(9), 1954-1961.
- Glaessgen, E., & Stargel, D. (2012). The Digital Twin Paradigm for Future NASA and US Air Force Vehicles. 4th International Conference on System Engineering and Modeling (ICSEM).
- Goodier, C. I., & Gibb, A. G. F. (2003). Future opportunities for offsite construction. Building Research & Information, 31(2), 85-94.
- Hammerstrom, D. J. (2007). Metering for smart energy networks. Springer Science & Business Media.
- Irizarry, J., Gheisari, M., & Walker, B. N. (2013). Automating site safety inspections using ontologies and unmanned aerial vehicles. Journal of Computing in Civil Engineering, 27(1), 1-13.
- ISO 14040. (2006). Environmental management–Life cycle assessment–Principles and framework. International Organization for Standardization.
- Jardine, A. K. S., Lin, D., & Banjevic, D. (2006). A review on machinery diagnostics and prognostics implementing condition-based maintenance. Mechanical Systems and Signal Processing, 20(7), 1483-1526.
- Jordan, M. I., & Mitchell, T. M. (2015). Machine learning: Trends, perspectives, and prospects. Science, 349(6245), 255-260.
- Kolarevic, B. (2003). Architecture in the digital age: Design and manufacturing. Taylor & Francis.
- Lim, S., Buswell, R. A., Le, T. T., Austin, R. J., Gibb, A. G. F., & Thorpe, T. (2012). Developments in construction-scale additive manufacturing processes. Automation in Construction, 21, 262-268.
- Manyika, J., Chui, M., Miremadi, M., Bughin, J., Allas, T., Dahlström, P., … & Woetzel, J. (2017). A future that works: Automation, employment, and productivity. McKinsey Global Institute.
- Meyers, M. A., & Chawla, K. K. (2009). Mechanical behavior of materials. Cambridge university press.
- O’Neil, C. (2016). Weapons of math destruction: How big data increases inequality and threatens democracy. Crown.
- OECD. (2017). Regulatory policy and governance: Supporting economic growth and serving the public interest. OECD Publishing.
- Rutten, D. (2007). Grasshopper: Visual programming in 3D. 3D Modeling and Design.
- Swan, M. (2015). Blockchain: Blueprint for a new economy. O’Reilly Media, Inc.
- USGBC. (2023). LEED. https://www.usgbc.org/leed
- Weber, R. H. (2015). Internet of Things–New security and privacy challenges. Computer Law & Security Review, 31(3), 321-331.
- Whyte, J. (2002). Virtual reality and the built environment. Architectural Press.
So, buildings might start designing themselves with AI? Finally, my dream of a self-assembling LEGO house might actually come true. Just need to figure out how to avoid the AI Skynet scenario when my house decides it needs a moat…and an army of robot butlers.
That’s a fun thought! The idea of AI handling design opens up some interesting possibilities. Perhaps future building codes will include ‘ethics protocols’ for AI-designed structures to prevent any moat-related incidents. The possibilities are endless!
Editor: FocusNews.Uk
Thank you to our Sponsor Focus 360 Energy
AI architects, you say? I look forward to my robot overlords designing energy-efficient dog houses. Let’s hope their algorithms factor in proper belly-rub access and strategically placed treat dispensers!
That’s a great point! The idea of AI designing for specific needs, even for our furry friends, is fascinating. Imagine algorithms that personalize living spaces based on individual animal behaviors and preferences. We could see a revolution in pet-friendly architecture! Thanks for the fun thought!
Editor: FocusNews.Uk
Thank you to our Sponsor Focus 360 Energy
AI-designed buildings? Fantastic! Just imagine the possibilities… self-cleaning windows that also judge your interior decorating skills! I look forward to my future home gently suggesting I donate that lava lamp to charity.