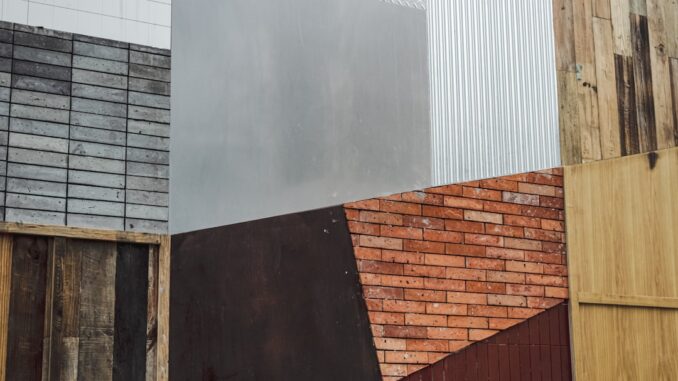
Abstract
The building envelope serves as a crucial interface between the interior environment and the external climate, significantly influencing energy consumption, occupant comfort, and overall building performance. This report provides a comprehensive examination of the building envelope, moving beyond basic energy efficiency considerations to encompass a holistic perspective. It delves into the fundamental principles of building science governing envelope behavior, including heat transfer mechanisms, air leakage, moisture management, and the synergistic interaction of various envelope components. The report explores the complexities of material science and engineering, assessing the performance characteristics of conventional and innovative building materials. Furthermore, it investigates advanced envelope designs, such as high-performance walls and roofs, vacuum insulated panels (VIPs), and aerogel composites, analyzing their potential for enhanced thermal resistance, reduced air infiltration, and improved moisture control. The integration of renewable energy systems, such as building-integrated photovoltaics (BIPV) and solar thermal collectors, within the envelope is also addressed. Finally, the report highlights the importance of life cycle assessment (LCA) and sustainability considerations in envelope design, emphasizing the need for environmentally responsible material selection and construction practices. The paper concludes with a discussion of future research directions and technological advancements that will shape the next generation of building envelopes, leading towards net-zero energy and resilient built environments.
Many thanks to our sponsor Focus 360 Energy who helped us prepare this research report.
1. Introduction
The building envelope, defined as the physical separator between the conditioned interior of a building and the unconditioned exterior environment, plays a paramount role in determining a building’s energy performance, indoor environmental quality, and long-term durability [1]. The envelope’s primary function is to moderate the flow of heat, air, and moisture, thereby minimizing energy consumption for heating, cooling, and ventilation while ensuring occupant comfort and preventing structural damage [2]. Traditionally, the focus has been on improving thermal resistance (R-value) through insulation and reducing air leakage through sealing. However, a comprehensive understanding of building science principles reveals that the envelope is a complex, dynamic system where various factors interact synergistically [3].
This report argues for a holistic approach to building envelope design, moving beyond prescriptive measures and embracing performance-based strategies that consider the specific climate, building type, occupancy patterns, and material properties. It emphasizes the importance of understanding the underlying physics and material science that govern envelope behavior and the need to integrate advanced technologies and sustainable practices into the design process. The report addresses challenges in envelope performance such as thermal bridging, moisture accumulation, and degradation of materials, exploring potential solutions and strategies to mitigate these risks. Furthermore, it assesses the role of building information modeling (BIM) and simulation tools in optimizing envelope design and predicting long-term performance.
Many thanks to our sponsor Focus 360 Energy who helped us prepare this research report.
2. Building Science Principles
2.1 Heat Transfer Mechanisms
Heat transfer through the building envelope occurs primarily through three mechanisms: conduction, convection, and radiation [4].
-
Conduction: Heat transfer through a solid material due to a temperature difference. The rate of conduction is proportional to the material’s thermal conductivity (k-value) and the temperature gradient. Materials with low thermal conductivity, such as insulation, are effective at reducing conductive heat transfer.
-
Convection: Heat transfer through the movement of fluids (air or water). Convection can occur naturally due to buoyancy effects (natural convection) or be forced by fans or pumps (forced convection). Air leakage through cracks and gaps in the envelope is a significant source of convective heat transfer, leading to energy loss and discomfort.
-
Radiation: Heat transfer through electromagnetic waves. All objects emit thermal radiation, and the amount of radiation emitted depends on the object’s temperature and emissivity. Surfaces with high emissivity, such as dark-colored materials, absorb and emit more radiant heat than surfaces with low emissivity, such as reflective materials. Solar radiation is a major source of radiant heat gain in buildings, particularly through windows and roofs.
The relative importance of each heat transfer mechanism depends on the specific envelope component, climate, and building orientation. For example, conduction is the dominant mode of heat transfer through insulated walls, while radiation is the dominant mode of heat transfer through windows exposed to direct sunlight.
2.2 Air Leakage and Infiltration
Air leakage, also referred to as infiltration when uncontrolled, is the unintentional movement of air through cracks, gaps, and other openings in the building envelope [5]. Air leakage can significantly increase energy consumption by allowing conditioned air to escape and unconditioned air to enter the building. It can also lead to moisture problems, such as condensation and mold growth, by carrying moisture-laden air into the building structure. Air leakage is quantified by the air changes per hour (ACH) and leakage area. Reducing air leakage is a critical aspect of improving building envelope performance. Strategies for minimizing air leakage include sealing cracks and gaps with caulk, weather stripping, and expanding foam; using air barriers to create a continuous airtight layer; and pressurizing the building to identify and seal leaks.
2.3 Moisture Management
Moisture management is a critical consideration in building envelope design to prevent moisture-related problems, such as rot, mold, corrosion, and deterioration of building materials [6]. Moisture can enter the building envelope through various pathways, including air leakage, diffusion, rainwater penetration, and ground moisture. Effective moisture management strategies involve controlling the sources of moisture, preventing moisture from entering the building envelope, and providing pathways for moisture to escape. Key principles of moisture management include:
-
Vapor diffusion control: Using vapor retarders or vapor barriers to limit the diffusion of water vapor through the building envelope.
-
Rainwater management: Designing roofs, walls, and windows to effectively shed rainwater and prevent water penetration.
-
Air leakage control: Minimizing air leakage to prevent moisture-laden air from entering the building envelope.
-
Drainage: Providing drainage pathways to allow moisture to escape from the building envelope.
The selection of appropriate moisture management strategies depends on the climate, building type, and construction materials. In cold climates, where inward vapor drive is dominant, vapor retarders are typically placed on the warm side of the insulation. In hot, humid climates, where outward vapor drive is dominant, vapor-permeable materials are preferred to allow moisture to escape. “Smart” vapor retarders are also now commonly used which vary their permeance with ambient humidity.
2.4 Interaction of Envelope Components
The building envelope is not simply the sum of its individual components; rather, it is a complex system where the performance of each component is influenced by its interaction with other components [7]. For example, the performance of a wall assembly depends on the thermal resistance, air leakage, and moisture management characteristics of the insulation, sheathing, cladding, and vapor retarder. Similarly, the performance of a window depends on the thermal properties of the glazing, frame, and sealant, as well as its installation quality and orientation. Therefore, a holistic approach to building envelope design requires careful consideration of the interaction of all envelope components. Key considerations include:
-
Thermal bridging: Minimizing thermal bridging, which occurs when a highly conductive material penetrates the insulation layer, creating a pathway for heat to flow through the envelope.
-
Air barrier continuity: Ensuring that the air barrier is continuous and airtight throughout the entire envelope.
-
Moisture compatibility: Selecting materials that are compatible with each other in terms of moisture permeability and preventing moisture accumulation within the envelope.
-
Structural integrity: Ensuring that the envelope is structurally sound and can withstand wind loads, snow loads, and other environmental stresses.
Many thanks to our sponsor Focus 360 Energy who helped us prepare this research report.
3. Material Science and Engineering
3.1 Conventional Building Materials
Conventional building materials, such as concrete, brick, wood, and glass, have been used for centuries in building construction. These materials have well-established properties, availability, and cost-effectiveness. However, they also have limitations in terms of thermal performance, durability, and environmental impact [8].
-
Concrete: Concrete is a strong and durable material, but it has relatively low thermal resistance and high thermal mass. Concrete walls can contribute to thermal bridging and condensation if not properly insulated.
-
Brick: Brick is a durable and aesthetically pleasing material, but it also has relatively low thermal resistance and high thermal mass. Brick walls require insulation to meet modern energy codes.
-
Wood: Wood is a renewable and sustainable material, but it is susceptible to moisture damage, decay, and insect infestation. Wood frame walls require proper moisture management and pest control.
-
Glass: Glass is a transparent material that allows natural light to enter the building, but it has relatively low thermal resistance and high solar heat gain. High-performance glazing, such as low-e coatings and insulated glazing units, can improve the thermal performance of windows.
3.2 Advanced Building Materials
Advanced building materials, such as high-performance insulation, aerogel, vacuum insulation panels, and phase change materials, offer improved thermal performance, durability, and sustainability compared to conventional materials [9].
-
High-performance insulation: High-performance insulation materials, such as spray foam, mineral wool, and rigid foam boards, have higher R-values per inch than conventional insulation materials, such as fiberglass and cellulose.
-
Aerogel: Aerogel is a highly porous material with extremely low thermal conductivity. Aerogel insulation can significantly reduce heat transfer through the building envelope, but it is currently expensive and requires careful installation.
-
Vacuum insulation panels (VIPs): VIPs consist of a rigid core material enclosed in a gas-tight envelope under vacuum. VIPs have extremely high thermal resistance and can be used in applications where space is limited, such as retrofits and appliance insulation.
-
Phase change materials (PCMs): PCMs absorb and release heat during phase transitions, such as melting and freezing. PCMs can be integrated into building materials to improve thermal mass and reduce temperature fluctuations.
The selection of appropriate building materials depends on the specific application, climate, budget, and sustainability goals. It is important to consider the life cycle cost and environmental impact of each material, as well as its performance characteristics.
3.3 Sustainable Materials
The use of sustainable building materials is becoming increasingly important as architects and engineers seek to reduce the environmental impact of buildings [10]. Sustainable building materials are those that are renewable, recycled, or locally sourced, and that have low embodied energy and low environmental impact. Examples of sustainable building materials include:
-
Recycled content materials: Materials made from recycled content, such as recycled steel, recycled glass, and recycled plastic.
-
Bio-based materials: Materials made from renewable biological resources, such as wood, bamboo, straw, and hemp.
-
Locally sourced materials: Materials that are extracted, processed, and manufactured locally, reducing transportation costs and environmental impact.
-
Durable materials: Materials that have a long service life and require minimal maintenance, reducing the need for replacement and repair.
Many thanks to our sponsor Focus 360 Energy who helped us prepare this research report.
4. Advanced Envelope Designs
4.1 High-Performance Walls and Roofs
High-performance walls and roofs are designed to minimize heat transfer, air leakage, and moisture accumulation, while providing structural support and aesthetic appeal [11]. These assemblies typically incorporate multiple layers of materials, including insulation, air barriers, vapor retarders, and cladding. Examples of high-performance wall and roof designs include:
-
Double-wall construction: A wall system with two separate stud walls, creating a wide cavity for insulation and reducing thermal bridging.
-
Structural insulated panels (SIPs): A composite panel consisting of a rigid foam core sandwiched between two layers of structural sheathing. SIPs provide high thermal resistance and structural strength.
-
Insulated concrete forms (ICFs): A concrete wall system formed by interlocking foam blocks, which provide insulation and formwork for the concrete.
-
Green roofs: Roofs covered with vegetation, which provide insulation, reduce stormwater runoff, and improve air quality.
4.2 Integration of Renewable Energy Systems
The building envelope can be integrated with renewable energy systems, such as building-integrated photovoltaics (BIPV) and solar thermal collectors, to generate electricity and heat [12]. BIPV systems consist of photovoltaic cells integrated into building materials, such as roofing tiles, wall panels, and windows. Solar thermal collectors use the building envelope to capture solar energy for heating water or air.
-
Building-integrated photovoltaics (BIPV): PV cells integrated into building materials, such as roofing tiles, wall panels, and windows.
-
Solar thermal collectors: Systems that use the building envelope to capture solar energy for heating water or air.
Integrating renewable energy systems into the building envelope can significantly reduce reliance on fossil fuels and lower energy costs. However, careful design and installation are required to ensure optimal performance and prevent moisture problems.
4.3 Smart Envelopes
Smart envelopes are adaptive building envelopes that can automatically adjust their thermal and optical properties in response to changing environmental conditions and occupant needs [13]. Smart envelopes can incorporate technologies such as electrochromic glazing, thermochromic coatings, and dynamic insulation. Electochromic glass darkens when a voltage is applied to it which can reduce solar heat gain and glare. Thermochromic coatings change color in response to temperature changes which can reduce the heat absorbed into the envelope. The dynamic insulation would adjust it R-value in response to heat flow. By intelligently managing heat transfer, solar gain, and daylighting, smart envelopes can significantly improve energy efficiency and occupant comfort.
Many thanks to our sponsor Focus 360 Energy who helped us prepare this research report.
5. Life Cycle Assessment and Sustainability
Life cycle assessment (LCA) is a method for evaluating the environmental impacts of a product or service throughout its entire life cycle, from raw material extraction to disposal [14]. LCA can be used to assess the environmental impacts of building envelope materials and designs, considering factors such as embodied energy, greenhouse gas emissions, and resource depletion. LCA is also used to investigate the environmental impacts, health and safety impacts, social impacts and economic impacts throughout the entire lifecycle of a product or service.
Sustainability considerations are becoming increasingly important in building envelope design, as architects and engineers seek to minimize the environmental impact of buildings and promote long-term durability and resilience. Sustainable envelope design involves selecting materials that are renewable, recycled, or locally sourced, and designing assemblies that minimize energy consumption, air leakage, and moisture accumulation. It is also important to consider the end-of-life disposal of envelope materials and to design for disassembly and reuse. The importance of durability cannot be overlooked from a sustainability perspective.
Many thanks to our sponsor Focus 360 Energy who helped us prepare this research report.
6. Future Directions and Technological Advancements
The future of building envelope design will be shaped by several key trends and technological advancements [15]. These include:
-
Nanomaterials: Nanomaterials, such as aerogels and carbon nanotubes, offer the potential for ultra-high-performance insulation and lightweight structural materials.
-
3D printing: 3D printing technology can be used to create complex building envelope components with customized shapes and properties.
-
Advanced sensors and controls: Advanced sensors and controls can be used to monitor and optimize the performance of building envelopes in real-time.
-
BIM and simulation tools: Building information modeling (BIM) and simulation tools can be used to design and analyze building envelopes more effectively.
-
Development of new regulatory frameworks: New regulatory frameworks are needed to encourage the adoption of high-performance building envelopes and promote sustainable construction practices.
Research and development efforts should focus on these key areas to accelerate the development and deployment of innovative building envelope technologies.
Many thanks to our sponsor Focus 360 Energy who helped us prepare this research report.
7. Conclusion
The building envelope is a critical component of building performance, influencing energy consumption, occupant comfort, and long-term durability. A holistic approach to building envelope design requires a deep understanding of building science principles, material science and engineering, and sustainability considerations. Advanced envelope designs, such as high-performance walls and roofs, integrated renewable energy systems, and smart envelopes, offer the potential to significantly improve building performance and reduce environmental impact. Continued research and development efforts are needed to develop and deploy innovative envelope technologies that will lead to net-zero energy and resilient built environments.
Many thanks to our sponsor Focus 360 Energy who helped us prepare this research report.
References
[1] Straube, J. (2011). High-performance building envelopes: What, why and how. Building Science Corporation.
[2] Allen, J. K., & Iano, P. A. (2015). Fundamentals of building construction: Materials and methods. John Wiley & Sons.
[3] Lstiburek, J. (2008). Building science basics. Building Science Corporation.
[4] Incropera, F. P., DeWitt, D. P., Bergman, T. L., & Lavine, A. S. (2007). Fundamentals of heat and mass transfer. John Wiley & Sons.
[5] Walker, I. S., & Wilson, D. J. (1998). Air leakage performance of Canadian houses. ASHRAE Transactions, 104(1), 1345-1358.
[6] TenWolde, A., Rose, W. B., & Straube, J. F. (2007). Understanding and preventing decay in residential buildings. Forest Products Laboratory.
[7] Burnett, E. F. P. (2004). Thermal performance of buildings. Taylor & Francis.
[8] Minke, G. (2000). Building with earth: Design and technology of a sustainable architecture. Birkhäuser.
[9] Baetens, R., Jelle, B. P., & Gustavsen, A. (2011). Aerogel insulation for building applications: A state-of-the-art review. Energy and Buildings, 43(4), 761-769.
[10] Kibert, C. J. (2016). Sustainable construction: Green building design and delivery. John Wiley & Sons.
[11] U.S. Department of Energy. (2017). Building America Solution Center. https://basc.pnnl.gov/
[12] Luque, A., & Hegedus, S. (2011). Handbook of photovoltaic science and engineering. John Wiley & Sons.
[13] Loonen, R. C. G. M., Trčka, D., Cóstola, D., & Hensen, J. L. M. (2013). Climate adaptive building shells: State-of-the-art and future challenges. Renewable and Sustainable Energy Reviews, 25, 483-493.
[14] Curran, M. A. (2013). Life cycle assessment: Principles and practice. US Environmental Protection Agency.
[15] D’Agostino, D., Parker, D., & Diamond, R. (2016). Building energy innovations: A vision for U.S. Department of Energy’s building technologies office. Ernest Orlando Lawrence Berkeley National Laboratory.
Net-zero energy buildings? Sounds ambitious! Let’s hope those advanced sensors and controls mentioned actually talk to each other, and not just create a very expensive, energy-hogging light show.
That’s a valid point! The integration of sensors and controls is indeed critical. A fragmented system defeats the purpose. We must ensure seamless communication to truly optimize energy performance and avoid that dreaded ‘energy-hogging light show.’ Thanks for highlighting this important aspect!
Editor: FocusNews.Uk
Thank you to our Sponsor Focus 360 Energy
So, we’re aiming for net-zero with, like, *actual* sustainable materials this time? No more greenwashing where “sustainable” means “shipped from the other side of the planet,” right? Inquiring minds need to know.
That’s absolutely right! We’re focusing on locally sourced and truly sustainable materials. Reducing transportation is key to minimizing the environmental impact. We’re carefully evaluating the entire life cycle to avoid any ‘greenwashing’. Thanks for keeping us honest! Let’s push for genuine sustainability together.
Editor: FocusNews.Uk
Thank you to our Sponsor Focus 360 Energy
Given the emphasis on material science, could you elaborate on the practical challenges of integrating advanced materials like aerogels or vacuum insulation panels into existing building stock for retrofit projects?