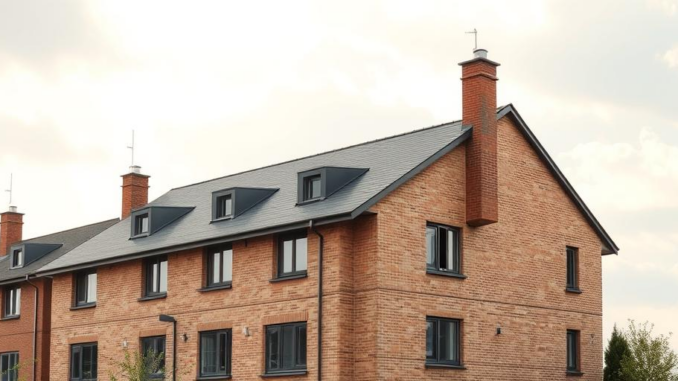
Abstract
Airtightness is a critical performance parameter in modern building design, impacting energy efficiency, indoor environmental quality (IEQ), and long-term building durability. While the benefits of airtight construction are well-documented, achieving and maintaining optimal airtightness levels presents significant challenges. This research report provides a comprehensive review of airtightness principles, examining various strategies for minimizing air leakage, advanced testing methodologies, and the synergistic relationship between airtightness and other building performance factors. It delves into the materials science behind airtight building envelopes, exploring emerging technologies and innovative approaches for enhancing airtightness in both new construction and retrofit applications. Furthermore, this report critically assesses the current state of airtightness standards and regulations, identifies knowledge gaps, and proposes future research directions to advance the field and promote the widespread adoption of high-performance, airtight buildings.
Many thanks to our sponsor Focus 360 Energy who helped us prepare this research report.
1. Introduction
The built environment is a major consumer of energy, accounting for a substantial portion of global energy consumption and greenhouse gas emissions. Reducing the energy demand of buildings is therefore paramount in mitigating climate change and achieving sustainability goals. Airtightness, defined as the resistance of a building envelope to uncontrolled air leakage, plays a pivotal role in minimizing energy waste and optimizing building performance. Uncontrolled air leakage results in significant heat loss during the heating season and heat gain during the cooling season, leading to increased energy consumption for space conditioning. Furthermore, air leakage can compromise indoor air quality by allowing pollutants, allergens, and moisture to enter the building, potentially leading to health problems and building durability issues. This report examines the multifaceted aspects of airtightness, encompassing its theoretical underpinnings, practical implementation, testing methodologies, and its impact on various building performance parameters.
Many thanks to our sponsor Focus 360 Energy who helped us prepare this research report.
2. Principles of Airtightness
Airtightness is governed by fundamental principles of physics related to pressure differences, air flow, and material properties. Air leakage occurs when a pressure differential exists across the building envelope, driving air flow through unintentional gaps, cracks, and penetrations. These pressure differences can arise from various sources, including wind pressure, stack effect (buoyancy-driven air flow due to temperature differences), and mechanical ventilation systems. The rate of air leakage is influenced by the magnitude of the pressure difference, the size and shape of the openings, and the air permeability of the building materials. The air permeability is defined as the rate of air flow through a material per unit area per unit pressure difference, it is expressed in $m^3/(s.m^2.Pa)$ units.
Airtightness is often quantified using metrics such as air changes per hour at 50 Pascals (ACH50) and leakage area. ACH50 represents the number of times the entire volume of air inside a building is replaced per hour when a pressure difference of 50 Pascals is applied across the building envelope. Leakage area represents the effective size of all the air leakage pathways in the building envelope. These metrics are typically determined using a blower door test, a standardized procedure for measuring building airtightness. A low ACH50 value indicates a more airtight building, while a high value indicates a leakier building.
Furthermore, the location and distribution of air leakage pathways are critical factors affecting building performance. Air leakage concentrated in certain areas, such as around windows and doors, or in thermal bridges, can lead to localized discomfort and condensation problems. Understanding the airflow patterns and identifying the primary air leakage pathways is essential for effective airtightness improvement.
Many thanks to our sponsor Focus 360 Energy who helped us prepare this research report.
3. Strategies for Achieving Airtightness
Airtightness can be achieved through a combination of design strategies, material selection, and construction practices. A holistic approach that considers the entire building envelope is crucial for minimizing air leakage effectively. The following are some of the key strategies:
- Continuous Air Barrier: A continuous air barrier is a primary component of an airtight building envelope. It is designed to prevent air from flowing through the walls, roof, and foundation. Materials used for the air barrier must be airtight, durable, and compatible with other building materials. Common air barrier materials include membranes, liquid-applied coatings, and rigid insulation boards with sealed joints. Continuity is key, and careful attention must be paid to detailing around penetrations, such as windows, doors, and service entries.
- Sealing Gaps and Cracks: Gaps and cracks in the building envelope are major sources of air leakage. Sealing these openings is essential for improving airtightness. Common sealing methods include caulking, weather stripping, and the use of expanding foam. Caulking is typically used to seal gaps around windows, doors, and other building components. Weather stripping is used to create a tight seal between operable windows and doors and their frames. Expanding foam is used to fill larger gaps and cavities.
- Addressing Penetrations: Penetrations through the building envelope, such as pipes, wires, and ducts, create opportunities for air leakage. These penetrations must be carefully sealed to maintain airtightness. Specialized sealing products, such as pipe boots and wire chases, are available for this purpose. Ensuring that all penetrations are properly sealed during construction is crucial, as retrofitting these areas can be challenging and costly.
- Optimizing Building Envelope Design: The design of the building envelope plays a significant role in achieving airtightness. Simple, well-detailed building designs are generally easier to make airtight than complex, intricate designs. Minimizing the number of penetrations and thermal bridges can also improve airtightness. Careful consideration should be given to the selection of building materials and their compatibility with each other.
- Air Sealing Membranes: Air sealing membranes are used to create a continuous airtight layer within the building envelope. These membranes are typically made of polymer-based materials and are applied to the exterior or interior of the building’s structural components. They can be self-adhering or mechanically attached, depending on the application. Key considerations when selecting air sealing membranes include their air permeability, durability, and compatibility with other building materials.
- Liquid-Applied Air Barriers: Liquid-applied air barriers are coatings that are sprayed or rolled onto the building’s structural components to create an airtight layer. These barriers are typically made of elastomeric polymers and are designed to stretch and flex with the building’s movement. Liquid-applied air barriers are often used in complex building designs where it is difficult to install membranes.
- Airtight Windows and Doors: Windows and doors are often a significant source of air leakage in buildings. Selecting airtight windows and doors with low air leakage ratings is essential for achieving a high-performance building envelope. Key features to look for in airtight windows and doors include multiple layers of weather stripping, insulated frames, and tight-fitting sashes.
- Foundation Sealing: Foundations can be a significant source of air leakage, particularly in buildings with uninsulated or poorly sealed foundations. Sealing the foundation walls and slab can significantly improve building airtightness. Methods for sealing foundations include applying a waterproof membrane to the exterior of the foundation walls, sealing cracks and penetrations in the foundation slab, and insulating the foundation walls.
Many thanks to our sponsor Focus 360 Energy who helped us prepare this research report.
4. Techniques for Testing Airtightness
Measuring airtightness is essential for verifying the effectiveness of airtightness measures and identifying air leakage pathways. The following are some of the common techniques for testing airtightness:
- Blower Door Test: The blower door test is the most widely used method for measuring building airtightness. It involves installing a powerful fan in an exterior doorway to depressurize or pressurize the building. The fan measures the amount of air required to maintain a specific pressure difference across the building envelope. The resulting data is used to calculate metrics such as ACH50 and leakage area. Blower door tests can also be used to locate air leakage pathways by using smoke pencils or thermal imaging cameras to identify areas where air is entering or exiting the building.
- Smoke Pencil Test: Smoke pencils are used to visually detect air leakage pathways. A smoke pencil emits a small stream of smoke that is sensitive to air currents. By holding a smoke pencil near potential air leakage pathways, such as around windows, doors, and penetrations, one can identify areas where air is entering or exiting the building.
- Thermal Imaging: Thermal imaging cameras are used to detect temperature differences on the surface of building components. Air leakage can cause localized temperature changes, which can be detected by a thermal imaging camera. Thermal imaging can be used to identify air leakage pathways in walls, roofs, and foundations.
- Tracer Gas Techniques: Tracer gas techniques involve releasing a small amount of non-toxic gas into the building and measuring the concentration of the gas over time. The rate at which the gas dissipates from the building is an indicator of the building’s airtightness. Tracer gas techniques can be used to measure airtightness in large or complex buildings where it is difficult to conduct a blower door test.
- Acoustic Leak Detection: Acoustic leak detection methods use sensitive microphones to detect the sound of air leaking through the building envelope. These methods can be used to locate air leakage pathways in walls, roofs, and foundations.
Selection of the appropriate testing method depends on the size and complexity of the building, the objectives of the testing, and the available resources. Blower door tests are typically used for whole-building airtightness testing, while smoke pencils and thermal imaging can be used to locate specific air leakage pathways.
Many thanks to our sponsor Focus 360 Energy who helped us prepare this research report.
5. Impact of Airtightness on Building Performance
Airtightness has a significant impact on various aspects of building performance, including energy consumption, indoor air quality, and building durability. The following are some of the key impacts:
- Energy Consumption: Airtightness reduces energy consumption by minimizing air leakage, which is a major source of heat loss during the heating season and heat gain during the cooling season. Reduced air leakage leads to lower heating and cooling loads, resulting in significant energy savings. Airtightness also allows for more efficient operation of HVAC systems, as they do not have to compensate for uncontrolled air leakage. Many studies have shown a strong correlation between airtightness and energy performance, with tighter buildings exhibiting significantly lower energy consumption.
- Indoor Air Quality (IAQ): Airtightness can improve IAQ by preventing the infiltration of pollutants, allergens, and moisture into the building. Uncontrolled air leakage can introduce outdoor pollutants, such as pollen, dust, and vehicle exhaust, into the building, compromising IAQ. Airtightness also reduces the risk of moisture intrusion, which can lead to mold growth and other IAQ problems. However, it is important to ensure adequate ventilation in airtight buildings to remove indoor pollutants and maintain healthy IAQ. This is often achieved through mechanical ventilation systems with heat recovery, ensuring a controlled and energy-efficient exchange of air.
- Building Durability: Airtightness can improve building durability by preventing moisture intrusion and condensation. Moisture can damage building materials, such as wood and insulation, leading to rot, decay, and structural problems. Airtightness reduces the risk of moisture intrusion by preventing air leakage, which can carry moisture into the building envelope. Furthermore, airtightness helps to control condensation by preventing warm, moist air from contacting cold surfaces within the building envelope. An air tight layer also provides a degree of protection from pests entering the building from outside.
- Thermal Comfort: Airtightness contributes to improved thermal comfort by reducing drafts and maintaining consistent temperatures throughout the building. Air leakage can cause drafts, which can make occupants feel uncomfortable, even if the overall temperature is within a comfortable range. Airtightness also helps to maintain consistent temperatures by preventing heat loss and gain through the building envelope.
- Acoustic Performance: While not a primary focus, airtightness can contribute to improved acoustic performance by reducing the transmission of sound through the building envelope. Gaps and cracks in the building envelope can allow sound to travel more easily, leading to noise pollution inside the building. Airtightness helps to reduce sound transmission by sealing these gaps and cracks.
Many thanks to our sponsor Focus 360 Energy who helped us prepare this research report.
6. Advanced Materials and Technologies for Airtightness
Advancements in materials science and technology have led to the development of new and innovative solutions for achieving airtightness in buildings. The following are some of the emerging materials and technologies:
- Smart Membranes: Smart membranes are air barrier membranes that have the ability to adapt their permeability based on humidity levels. These membranes allow moisture to escape from the building envelope when humidity levels are high, preventing moisture accumulation and promoting drying. When humidity levels are low, the membranes become more airtight, preventing air leakage. This adaptive behavior can help to optimize building performance and durability.
- Aerogel Insulation: Aerogel is a highly porous material with exceptional thermal insulation properties. It can also be used as an airtightness layer when applied as a coating or incorporated into insulation panels. Aerogel insulation can significantly improve both the thermal performance and airtightness of building envelopes.
- Vacuum Insulation Panels (VIPs): VIPs are high-performance insulation panels that consist of a rigid core material encased in an airtight envelope under vacuum. The vacuum significantly reduces heat transfer through the panel, resulting in exceptional thermal insulation properties. VIPs can also contribute to airtightness by providing a continuous airtight layer when properly sealed and installed.
- Self-Sealing Membranes: Self-sealing membranes are air barrier membranes that have the ability to self-seal around penetrations, such as nails and screws. This feature eliminates the need for additional sealing measures around penetrations, simplifying the installation process and improving airtightness.
- Spray-Applied Air Barriers: Spray-applied air barriers are coatings that are sprayed onto the building’s structural components to create an airtight layer. These barriers are typically made of elastomeric polymers and are designed to stretch and flex with the building’s movement. Spray-applied air barriers can be applied to complex building designs where it is difficult to install membranes.
- Phase Change Materials (PCMs): While not directly related to airtightness, PCMs can improve building energy performance by storing and releasing thermal energy. PCMs can be incorporated into building materials, such as gypsum board and concrete, to improve their thermal mass and reduce temperature fluctuations. This can lead to reduced heating and cooling loads and improved energy efficiency. Integrating airtight construction with use of PCM can lead to more efficient building performance.
Many thanks to our sponsor Focus 360 Energy who helped us prepare this research report.
7. Airtightness Standards and Regulations
Airtightness standards and regulations are increasingly being adopted worldwide to promote energy efficiency and improve building performance. These standards typically specify minimum airtightness requirements for new buildings and major renovations. The following are some of the key airtightness standards and regulations:
- Passive House Standard: The Passive House standard is a voluntary building standard that requires extremely high levels of energy efficiency, including a very low air leakage rate (typically less than 0.6 ACH50). Passive House buildings are designed to minimize energy consumption for heating and cooling, resulting in significant energy savings.
- LEED (Leadership in Energy and Environmental Design): LEED is a green building rating system that recognizes and rewards buildings that are designed and constructed to be environmentally responsible and resource-efficient. LEED includes credits for airtightness, encouraging builders to minimize air leakage and improve building performance.
- International Energy Conservation Code (IECC): The IECC is a model energy code that is used by many states and local jurisdictions in the United States. The IECC includes mandatory airtightness requirements for new buildings, specifying maximum air leakage rates. The stringency of the IECC varies depending on the jurisdiction.
- European Union Energy Performance of Buildings Directive (EPBD): The EPBD sets minimum energy performance requirements for buildings in the European Union, including airtightness requirements. The EPBD requires member states to implement national building codes that meet or exceed the EPBD requirements.
The adoption of airtightness standards and regulations is driving innovation in building design and construction, leading to the development of more airtight and energy-efficient buildings. However, there is still a need for greater awareness and education about airtightness, as well as for more rigorous enforcement of existing standards.
Many thanks to our sponsor Focus 360 Energy who helped us prepare this research report.
8. Challenges and Future Directions
While significant progress has been made in the field of airtightness, several challenges remain. These include:
- Complexity of Building Design: Complex building designs can make it difficult to achieve airtightness. Intricate shapes, numerous penetrations, and unconventional building materials can create challenges for sealing the building envelope effectively.
- Quality of Construction: The quality of construction is a critical factor affecting airtightness. Even the best materials and designs can fail if they are not properly installed. Ensuring that builders are properly trained and that construction practices are followed rigorously is essential for achieving airtightness.
- Cost: Achieving high levels of airtightness can add to the initial cost of construction. However, the long-term energy savings and improved building durability can offset these costs over time. Furthermore, as airtightness technologies become more widely adopted, their costs are likely to decrease.
- Maintaining Airtightness Over Time: Building materials can degrade over time, leading to cracks and gaps that can compromise airtightness. Regular inspections and maintenance are necessary to ensure that airtightness is maintained over the lifespan of the building.
- Integration with Ventilation: Airtightness must be carefully integrated with ventilation strategies to ensure that buildings have adequate fresh air supply. Mechanical ventilation systems with heat recovery are often used in airtight buildings to provide controlled and energy-efficient ventilation.
Future research directions in the field of airtightness include:
- Development of new and improved airtightness materials: Research is needed to develop new materials that are more durable, easier to install, and more cost-effective.
- Development of advanced testing methods: New testing methods are needed to accurately measure airtightness in large and complex buildings.
- Development of building performance simulation tools: Detailed modelling of airflow in buildings linked to the building’s control systems is important to enhance airtightness.
- Improved understanding of the relationship between airtightness and IAQ: Further research is needed to better understand the impact of airtightness on IAQ and to develop strategies for optimizing both airtightness and IAQ.
- Development of strategies for retrofitting existing buildings: Retrofitting existing buildings to improve airtightness can be challenging and costly. Research is needed to develop more cost-effective and practical strategies for retrofitting existing buildings.
Many thanks to our sponsor Focus 360 Energy who helped us prepare this research report.
9. Conclusion
Airtightness is a crucial performance parameter in modern building design, impacting energy efficiency, indoor air quality, and building durability. Achieving and maintaining optimal airtightness levels requires a holistic approach that considers design strategies, material selection, construction practices, and testing methodologies. While challenges remain, advancements in materials science and technology, coupled with the increasing adoption of airtightness standards and regulations, are driving innovation and progress in the field. Future research efforts should focus on developing new materials and technologies, improving testing methods, and gaining a better understanding of the relationship between airtightness and other building performance parameters. By advancing the field of airtightness, we can create more energy-efficient, healthy, and durable buildings that contribute to a more sustainable future.
Many thanks to our sponsor Focus 360 Energy who helped us prepare this research report.
References
- American Society of Heating, Refrigerating and Air-Conditioning Engineers (ASHRAE). (2019). ASHRAE Standard 90.1-2019: Energy Standard for Buildings Except Low-Rise Residential Buildings. Atlanta, GA: ASHRAE.
- Building Science Corporation. (n.d.). Understanding Air Barriers. Retrieved from https://www.buildingscience.com/
- European Commission. (2010). Energy Performance of Buildings Directive (EPBD). Brussels, Belgium: European Commission.
- International Code Council (ICC). (2021). International Energy Conservation Code (IECC). Washington, D.C.: ICC.
- Persily, A., & Emmerich, S. J. (2015). Impact of airtightness on residential ventilation performance. Building and Environment, 94(Part 2), 786-794.
- Straube, J. (2011). High-Performance Enclosures. Building Science Press.
- Walker, I., & Sherman, M. (2005). Measured infiltration rates and implications for energy and IAQ performance. ASHRAE Transactions, 111(2), 363-375.
The report mentions the challenges in maintaining airtightness over time due to material degradation. What innovative solutions are being explored to create self-healing or adaptable building envelope materials that can automatically address breaches in airtightness?
That’s a great question! Self-healing polymers and bio-based materials that can respond to environmental changes are showing real promise. Further research is needed, but the potential for enhanced building longevity and reduced maintenance is huge. Let’s discuss more about the materials!
Editor: FocusNews.Uk
Thank you to our Sponsor Focus 360 Energy
So, achieving airtightness is crucial, but what about the poor building occupants trapped inside? Are we also researching the psychological effects of living in hermetically sealed boxes? Or are we just optimizing for energy efficiency at the expense of human well-being?