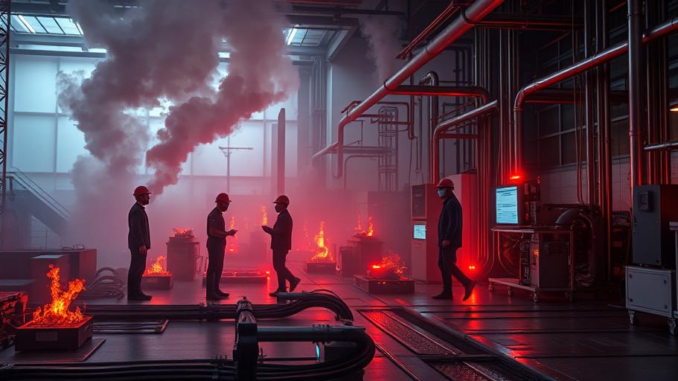
Abstract
Heat pump technology is experiencing a resurgence as a critical component of decarbonization strategies for buildings. While conventional air-source and ground-source heat pumps are increasingly prevalent, this report delves into the broader landscape of advanced heat pump systems, focusing on integration, optimization, and future directions. We examine transcritical CO2 heat pumps for high-temperature applications, absorption heat pumps utilizing waste heat, and hybrid systems that combine heat pumps with other renewable energy sources. Furthermore, we analyze advanced control strategies, refrigerant alternatives, and the potential for heat pumps to contribute to grid stabilization through demand response. This report aims to provide a comprehensive overview for experts in the field, highlighting the technical challenges, research opportunities, and policy considerations necessary to unlock the full potential of heat pump technology.
Many thanks to our sponsor Focus 360 Energy who helped us prepare this research report.
1. Introduction
The pressing need to mitigate climate change has spurred significant interest in reducing greenhouse gas (GHG) emissions from the building sector. Heating, ventilation, and air conditioning (HVAC) systems account for a substantial portion of building energy consumption, making them a key target for improvement. Heat pumps have emerged as a promising alternative to traditional fossil fuel-based heating systems, offering improved energy efficiency and the ability to leverage renewable electricity sources. The FHS (presumably a Framework or Scheme mentioned in the prompt) rightly identifies heat pumps as low-carbon heating solutions. However, the deployment of heat pump technology necessitates a deeper understanding of its capabilities, limitations, and potential for further advancement. This report expands upon the conventional understanding of heat pumps by exploring advanced system designs, novel refrigerants, and intelligent control strategies, aiming to provide a comprehensive perspective on the current state-of-the-art and future directions in the field.
Many thanks to our sponsor Focus 360 Energy who helped us prepare this research report.
2. Overview of Heat Pump Technology
At their core, heat pumps operate on the principle of transferring heat from a cooler source to a warmer sink. This is achieved through a thermodynamic cycle involving a refrigerant, a compressor, and two heat exchangers (evaporator and condenser). The choice of refrigerant significantly impacts the performance and environmental impact of the system. Traditional refrigerants like hydrochlorofluorocarbons (HCFCs) and hydrofluorocarbons (HFCs) have been phased out or are being phased down due to their ozone depletion potential (ODP) and global warming potential (GWP), respectively [1]. Newer refrigerants, including hydrofluoroolefins (HFOs), natural refrigerants (e.g., CO2, ammonia, propane), and low-GWP HFC blends are gaining prominence.
Heat pumps can be classified based on the heat source and sink. Air-source heat pumps (ASHPs) extract heat from the ambient air, while ground-source heat pumps (GSHPs), also known as geothermal heat pumps, utilize the relatively stable temperature of the ground. Water-source heat pumps (WSHPs) extract heat from water bodies. ASHPs are generally less expensive to install but their performance is significantly affected by ambient air temperature. GSHPs offer higher efficiency and more stable performance, but require a more complex and expensive installation process.
Energy efficiency is typically measured using the Coefficient of Performance (COP) for heating and the Energy Efficiency Ratio (EER) or Seasonal Energy Efficiency Ratio (SEER) for cooling. Higher COP, EER, and SEER values indicate better energy performance. Seasonal performance factors, such as the Seasonal Coefficient of Performance (SCOP) and Seasonal Energy Efficiency Ratio (SEER), provide a more realistic representation of the system’s performance over an entire heating or cooling season [2]. These metrics are important but can be misleading if used to only compare different ASHP, GSHP and WSHP because each have different operating characteristics.
Many thanks to our sponsor Focus 360 Energy who helped us prepare this research report.
3. Advanced Heat Pump Systems
While conventional ASHPs and GSHPs have established their place in the market, several advanced heat pump technologies offer unique advantages for specific applications.
3.1 Transcritical CO2 Heat Pumps
Transcritical CO2 (R744) heat pumps are gaining attention for their ability to deliver high-temperature water (up to 90°C) efficiently [3]. CO2 operates in a transcritical cycle, where the heat rejection process occurs above its critical point. This allows for efficient heat transfer even at high temperatures, making transcritical CO2 heat pumps suitable for district heating, industrial process heating, and domestic hot water applications where high temperatures are required. The advantages of CO2 as a refrigerant include its low GWP (GWP=1), non-flammability, and availability. However, CO2 heat pumps typically operate at higher pressures than conventional heat pumps, requiring specialized components and design considerations.
3.2 Absorption Heat Pumps
Absorption heat pumps (AHPs) utilize a thermodynamic cycle that relies on heat-driven absorption and desorption processes rather than mechanical compression. They use a working fluid pair, such as ammonia/water or lithium bromide/water. AHPs can be powered by waste heat from industrial processes, solar thermal energy, or natural gas, making them a promising technology for waste heat recovery and combined heat and power (CHP) applications [4]. The COP of AHPs is typically lower than that of vapor compression heat pumps, but their ability to utilize waste heat can significantly improve overall system efficiency and reduce reliance on electricity. AHPs are not widely installed because of their complexity and high initial cost. The COP is relatively low and requires special expertise to deploy.
3.3 Thermoelectric Heat Pumps
Thermoelectric heat pumps (TEHPs) operate on the Peltier effect, where heat is transferred when an electric current passes through a junction of two dissimilar materials. TEHPs are solid-state devices with no moving parts, offering advantages in terms of reliability and compactness. However, the COP of TEHPs is generally low compared to vapor compression heat pumps and AHPs [5]. TEHPs are best suited for niche applications requiring precise temperature control, such as cooling of electronic components, medical devices, and laboratory equipment. Recent advances in thermoelectric materials are improving the performance of TEHPs, but further research is needed to make them competitive with other heat pump technologies for widespread heating and cooling applications. In applications where vibration is to be avoided such as in a physics experiment, a TEHP can be deployed effectively.
3.4 Hybrid Heat Pump Systems
Hybrid heat pump systems combine heat pumps with other heating technologies, such as gas furnaces, boilers, or solar thermal systems. This approach allows for optimizing system performance under varying operating conditions. For example, a hybrid system might use a heat pump as the primary heating source during mild weather and switch to a gas furnace during extremely cold weather to maintain heating capacity and efficiency [6]. Hybrid systems can also integrate heat pumps with thermal energy storage, allowing for demand response and grid stabilization. The control strategy for hybrid systems is crucial to achieving optimal performance. Advanced control algorithms can predict heating demand, optimize energy source selection, and minimize operating costs. Another approach to hybrid heat pumps are systems that can be used for both Air Conditioning and providing domestic Hot water. In these systems, the heat is rejected to the domestic hot water tank thereby increasing the overall efficiency of the system. These systems tend to be expensive to install.
Many thanks to our sponsor Focus 360 Energy who helped us prepare this research report.
4. Optimization Strategies for Heat Pump Performance
Optimizing heat pump performance requires a multifaceted approach, encompassing component design, system integration, and control strategies.
4.1 Advanced Control Strategies
Advanced control strategies play a critical role in maximizing heat pump efficiency and minimizing energy consumption. Model Predictive Control (MPC) can be used to predict future heating and cooling demand based on weather forecasts, occupancy schedules, and building characteristics. MPC can then optimize heat pump operation to minimize energy costs while maintaining thermal comfort [7]. Fuzzy logic control and neural networks can be used to adapt heat pump operation to changing conditions and learn from past performance, further improving energy efficiency. Demand response capabilities can be integrated into heat pump control systems, allowing them to respond to grid signals and reduce electricity consumption during peak demand periods.
4.2 Heat Exchanger Design
Heat exchangers are a critical component of heat pump systems, affecting both performance and cost. Microchannel heat exchangers offer high heat transfer coefficients and compact designs, but are susceptible to fouling and require careful refrigerant distribution. Plate heat exchangers provide high efficiency and are suitable for water-to-water applications, but can be expensive and require large pressure drops [8]. Advanced heat exchanger designs, such as enhanced surface geometries and micro-fin tubes, can improve heat transfer and reduce pressure drop, leading to higher overall system efficiency. Research into new materials with improved thermal conductivity and corrosion resistance can further enhance heat exchanger performance.
4.3 Refrigerant Selection
The selection of a refrigerant is a critical decision in heat pump design, balancing performance, environmental impact, safety, and cost. HFOs offer low GWP and good thermodynamic properties but are flammable and can decompose into persistent trifluoroacetic acid (TFA) in the atmosphere [9]. Natural refrigerants such as CO2, ammonia, and propane offer low GWP and excellent thermodynamic properties but pose safety concerns due to toxicity or flammability. Low-GWP HFC blends can offer a compromise between performance, safety, and environmental impact, but their long-term availability and cost remain uncertain. Research into new refrigerants with improved properties is ongoing, focusing on developing stable, non-toxic, non-flammable, and environmentally benign alternatives.
4.4 System Integration and Configuration
The way a heat pump is integrated into a building’s HVAC system can significantly affect its performance. Proper sizing of the heat pump to match the building’s heating and cooling load is essential to avoid oversizing or undersizing. Ductwork design and insulation play a critical role in minimizing heat loss and maximizing airflow. Proper installation and commissioning are essential to ensure that the heat pump operates efficiently and reliably. System configuration, such as the use of variable speed compressors and electronic expansion valves, can further improve performance and energy efficiency. Furthermore, integration with smart home systems and energy management systems allows for remote monitoring and control, enabling optimization of energy consumption and demand response capabilities. Heat pump systems should be installed by certified technicians that are specifically trained for the equipment being installed. The configuration should also include a filter to ensure that large particulate matter does not enter the system thereby reducing efficiency.
Many thanks to our sponsor Focus 360 Energy who helped us prepare this research report.
5. Environmental Impacts and Sustainability
Heat pumps offer significant potential to reduce greenhouse gas emissions compared to traditional heating systems, particularly when powered by renewable electricity sources. The overall environmental impact of heat pump technology depends on several factors, including the energy efficiency of the heat pump, the carbon intensity of the electricity grid, and the leakage rate and GWP of the refrigerant. Life cycle assessments (LCAs) can be used to evaluate the environmental impacts of heat pump systems, considering all stages from manufacturing to disposal [10].
The use of low-GWP refrigerants is essential to minimize the direct environmental impact of heat pump technology. Proper handling and disposal of refrigerants are crucial to prevent leakage and release into the atmosphere. Policies and regulations are needed to encourage the adoption of low-GWP refrigerants and ensure responsible refrigerant management. Incentives and rebates can encourage the installation of high-efficiency heat pumps and promote the use of renewable electricity sources.
Furthermore, the integration of heat pumps with thermal energy storage can improve grid stability and reduce reliance on fossil fuel-based power plants. Demand response programs can incentivize consumers to shift their electricity consumption to off-peak hours, reducing peak demand and promoting the use of renewable energy. The overall sustainability of heat pump technology depends on a holistic approach that considers energy efficiency, refrigerant selection, system integration, and policy considerations.
Many thanks to our sponsor Focus 360 Energy who helped us prepare this research report.
6. Challenges and Opportunities
Despite the significant potential of heat pump technology, several challenges need to be addressed to facilitate its widespread adoption.
6.1 Cold Climate Performance
ASHPs can experience a significant drop in performance in cold climates, requiring supplemental heating or more advanced system designs. Research is needed to develop ASHPs with improved cold climate performance, such as multi-stage compressors, vapor injection cycles, and defrost strategies [11]. GSHPs offer more stable performance in cold climates but require a more expensive and complex installation process. Innovative ground heat exchanger designs and installation techniques can reduce the cost of GSHPs and make them more competitive with ASHPs.
6.2 Cost and Affordability
The initial cost of heat pump systems can be a barrier to adoption, particularly for low-income households. Government incentives and rebates can help to reduce the upfront cost and make heat pumps more affordable. Mass production and standardization of heat pump components can also help to lower costs. Innovative financing models, such as energy service agreements (ESAs), can allow consumers to pay for heat pump systems through energy savings, reducing the financial burden of upfront costs. The cost of installing GSHP can also be reduced through more efficient installation methods. GSHP boreholes can be bored using new technology such as directional drilling and can be cheaper than the traditional methods of vertically drilling a borehole.
6.3 Grid Integration
The widespread adoption of heat pumps can place a significant strain on the electricity grid, particularly during peak demand periods. Smart grid technologies and demand response programs are needed to manage the increased electricity demand and ensure grid stability. Virtual power plants (VPPs) can aggregate the capacity of multiple heat pumps and provide grid services, such as frequency regulation and load balancing. Energy storage technologies, such as batteries and thermal energy storage, can further improve grid integration and reduce reliance on fossil fuel-based power plants. The cost of batteries are falling every year as technology develops, this allows heat pumps to be paired with batteries at home or community level.
6.4 Public Awareness and Education
Lack of public awareness and understanding of heat pump technology can hinder its adoption. Education and outreach programs are needed to inform consumers about the benefits of heat pumps, including their energy efficiency, environmental advantages, and cost savings. Training programs for HVAC contractors and installers are essential to ensure that heat pump systems are properly installed and maintained. Clear and consistent labeling of heat pump performance and energy efficiency can help consumers make informed decisions. The public need to understand that a heat pump will not provide the same response as say a gas boiler. Education on operating characteristics is therefore paramount.
Many thanks to our sponsor Focus 360 Energy who helped us prepare this research report.
7. Future Directions
The future of heat pump technology is promising, with several emerging trends and research opportunities.
7.1 Smart and Connected Heat Pumps
Smart and connected heat pumps will be integrated into the Internet of Things (IoT), enabling remote monitoring, control, and optimization. Machine learning algorithms can be used to analyze data from heat pumps and optimize their performance based on real-time conditions. Predictive maintenance can be used to identify potential problems before they occur, reducing downtime and improving reliability. Integration with smart home systems and energy management systems will allow consumers to control their heating and cooling systems more effectively and reduce energy consumption. Smart thermostats will be able to learn user preferences and automatically adjust temperature settings to maximize comfort and energy savings.
7.2 Heat Pumps as Thermal Batteries
Heat pumps can be used to charge thermal energy storage systems, such as water tanks or phase change materials (PCMs), acting as thermal batteries. This allows for storing excess renewable energy and releasing it when needed, improving grid stability and reducing reliance on fossil fuel-based power plants. Thermal energy storage can also be used to shift electricity consumption to off-peak hours, reducing peak demand and lowering energy costs. Combining heat pumps with thermal batteries can provide a reliable and cost-effective solution for heating and cooling buildings, particularly in areas with high renewable energy penetration.
7.3 Heat Pumps for District Heating and Cooling
Heat pumps can be used to provide district heating and cooling services, improving energy efficiency and reducing greenhouse gas emissions at a community level. Centralized heat pump systems can utilize waste heat from industrial processes, data centers, or sewage treatment plants, reducing energy consumption and lowering operating costs. District heating and cooling networks can also integrate renewable energy sources, such as solar thermal and geothermal energy, further improving their sustainability. The deployment of heat pumps for district heating and cooling requires careful planning and coordination, considering factors such as building density, heat demand profiles, and grid infrastructure.
7.4 Advanced Materials and Manufacturing
Advances in materials science and manufacturing technologies can lead to improved heat pump performance and reduced costs. Nanomaterials and coatings can be used to enhance heat transfer and reduce corrosion. Additive manufacturing (3D printing) can be used to create complex heat exchanger geometries and customize heat pump components. High-efficiency compressors and motors can reduce energy consumption and improve overall system efficiency. Advanced sensors and controls can enable more precise monitoring and optimization of heat pump operation. Research into new materials and manufacturing processes is crucial to drive innovation and make heat pump technology more competitive.
Many thanks to our sponsor Focus 360 Energy who helped us prepare this research report.
8. Conclusion
Heat pump technology is a cornerstone of decarbonizing the building sector, and its potential extends far beyond traditional applications. By embracing advanced system designs, optimizing performance through intelligent control and innovative components, and addressing challenges related to cold climates, cost, and grid integration, we can unlock the full potential of heat pumps. Continued research, policy support, and public awareness are essential to accelerate the adoption of heat pump technology and create a more sustainable and resilient energy future. Focusing on public awareness is very important because the general public has little knowledge about heat pumps.
Many thanks to our sponsor Focus 360 Energy who helped us prepare this research report.
References
[1] IPCC, 2021: Climate Change 2021: The Physical Science Basis. Contribution of Working Group I to the Sixth Assessment Report of the Intergovernmental Panel on Climate Change [Masson-Delmotte, V., P. Zhai, A. Pirani, S.L. Connors, C. Péan, S. Berger, N. Caud, Y. Chen, L. Goldfarb, M.I. Gomis, M. Huang, K. Leitzell, E. Lonnoy, J.B.R. Matthews, T.K. Maycock, T. Waterfield, O. Yelekçi, R. Yu, and B. Zhou (eds.)]. Cambridge University Press, Cambridge, United Kingdom and New York, NY, USA, 2391 pp. doi:10.1017/9781009157896
[2] European Committee for Standardization (CEN). (2016). EN 14825:2016. Air conditioners, liquid chilling packages and heat pumps, with electrically driven compressors, for space heating and cooling – Testing and rating at part load conditions and calculation of seasonal performance.
[3] Lorentzen, G. (1994). Revival of carbon dioxide as a refrigerant. International Journal of Refrigeration, 17(5), 291-297.
[4] Herold, K. E., Radermacher, R., Klein, S. A., & Nellis, G. F. (2016). Absorption chillers and heat pumps. CRC press.
[5] Riffat, S. B., & Ma, X. (2003). Thermoelectrics: a review of present and potential applications. Applied Thermal Engineering, 23(8), 913-935.
[6] Hepbasli, A., & Akdemir, O. A. (2004). Thermodynamic performance assessment of a ground-source heat pump system. Energy Conversion and Management, 45(10), 1607-1627.
[7] Afram, A., & Janabi-Sharifi, F. (2014). Theory and applications of hierarchical model predictive control (MPC) in HVAC systems. Building and Environment, 72, 343-355.
[8] Shah, R. K., Sekulić, D. P., & Kline, S. J. (2003). Fundamentals of heat exchanger design. John Wiley & Sons.
[9] Sulbaek Andersen, M. P., Nielsen, O. J., Wallington, T. J., & Hurley, M. D. (2021). Atmospheric chemistry of unsaturated fluorocarbons: Lifetimes, removal processes, and products. Chemical Reviews, 121(1), 348-414.
[10] Reap, J., Roman, F., Duncan, S., & Bras, B. (2008). A survey of unresolved problems in life cycle assessment–Part 1: Goal and scope, data inventory, impact assessment, and interpretation. The International Journal of Life Cycle Assessment, 13(4), 290-300.
[11] O’Neal, D. L., Peterson, S., Abbaspour, M., & Ryu, H. (2011). Laboratory testing of air-source heat pumps at low ambient temperatures. ASHRAE Transactions, 117(1).
Given the variability in electricity grid carbon intensity, how might real-time data on grid emissions be integrated into heat pump control systems to further minimize their environmental impact?
That’s a great point! Integrating real-time grid emissions data is crucial. Imagine heat pumps optimizing their operation to coincide with periods of higher renewable energy availability on the grid. This could involve temporarily reducing heating during peak carbon intensity and pre-heating when cleaner energy is abundant. Thanks for highlighting this important consideration!
Editor: FocusNews.Uk
Thank you to our Sponsor Focus 360 Energy
The discussion of hybrid systems is particularly interesting. Has anyone explored the feasibility of integrating heat pumps with existing natural gas infrastructure on a community level, potentially using the gas network for peak demand periods or as a supplemental heat source in colder climates?