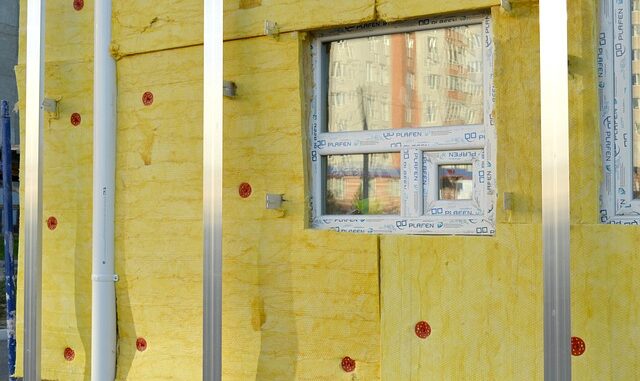
A Comprehensive Review of Thermal Resistance (R-value) and Its Role in Building Performance: Present Status, Limitations, and Future Directions
Abstract
Thermal resistance, commonly quantified by the R-value, is a cornerstone metric for evaluating the thermal performance of building envelopes. While widely adopted, its direct application and interpretation present complexities, particularly in the context of dynamic thermal conditions, advanced building materials, and holistic energy performance assessments. This review critically examines the current standards and methodologies used for R-value determination, explores the material-specific variations and their underlying physical mechanisms, and analyzes the influence of climate zones and building design on its effectiveness. Furthermore, it delves into the limitations of R-value as a sole indicator of insulation performance, including its inability to fully capture the effects of thermal bridging, air infiltration, and radiative heat transfer. Finally, the review considers potential future developments in insulation performance metrics, such as dynamic R-values, integrated thermal performance indicators, and advanced simulation tools, and the crucial role these advances will play in achieving ambitious energy efficiency targets for the built environment.
1. Introduction
The global imperative to reduce energy consumption in buildings has positioned thermal insulation as a critical element in building design and construction. Thermal resistance, universally represented by the R-value (or RSI-value in SI units), is the most prevalent measure of insulation’s ability to impede heat flow. A higher R-value signifies a greater resistance to heat transfer, theoretically translating to reduced energy consumption for heating and cooling. However, the simplistic nature of the R-value belies the complex reality of heat transfer mechanisms within building envelopes. While R-value serves as a valuable benchmark, its limitations in accurately predicting real-world energy performance are becoming increasingly apparent, especially as building designs become more sophisticated and materials become more advanced. This review aims to provide a critical assessment of R-value, exploring its fundamental principles, measurement methodologies, limitations, and potential future directions in the context of advanced building science and energy efficiency.
2. Fundamentals of R-value and Heat Transfer Mechanisms
At its core, R-value quantifies the resistance to conductive heat transfer through a material of a specified thickness. It is defined as the temperature difference required to drive a unit of heat flow through a unit area of the material for a unit of time. Mathematically, R = ΔT / q, where R is the thermal resistance, ΔT is the temperature difference, and q is the heat flux. The R-value is directly proportional to the material’s thickness and inversely proportional to its thermal conductivity (λ). Therefore, R = thickness / λ.
However, conductive heat transfer is only one piece of the puzzle. Buildings also experience heat transfer via convection and radiation. Convection involves heat transfer through the movement of fluids (air or liquid), while radiation involves the transfer of heat through electromagnetic waves. The relative importance of these three heat transfer modes depends on factors such as temperature differences, material properties, and the presence of air gaps. In many insulation materials, all three modes are present and interacting.
For example, fibrous insulation materials such as fiberglass and mineral wool rely on trapping air within their structure to reduce convective heat transfer. The solid fibers themselves contribute to conductive heat transfer, but the air pockets are designed to minimize air movement. Radiative heat transfer is also a factor, especially at higher temperatures. Similarly, rigid foam insulations such as expanded polystyrene (EPS) and extruded polystyrene (XPS) rely on closed cells filled with gas (often air or a blowing agent) to minimize conductive and convective heat transfer. Reflective insulation materials, such as radiant barriers, are designed to reduce radiative heat transfer by reflecting infrared radiation away from the building.
3. Standards and Methodologies for R-value Determination
The accurate and consistent determination of R-values is crucial for ensuring fair comparisons between different insulation materials and for predicting the thermal performance of building envelopes. Several standardized test methods are used to measure R-value, each with its own advantages and limitations.
-
ASTM C518 (Standard Test Method for Steady-State Thermal Transmission Properties by Means of the Heat Flow Meter Apparatus): This is one of the most widely used methods for measuring R-value. It involves placing a sample of the insulation material between two plates maintained at different temperatures. A heat flow meter measures the heat flux through the sample, and the R-value is calculated based on the temperature difference and the heat flux. ASTM C518 is suitable for measuring the R-value of relatively flat and uniform materials under steady-state conditions.
-
ASTM C177 (Standard Test Method for Steady-State Heat Flux Measurements and Thermal Transmission Properties by Means of the Guarded-Hot-Plate Apparatus): This method is similar to ASTM C518, but it uses a guarded hot plate to minimize lateral heat losses from the edges of the sample. This makes it more accurate for measuring the R-value of materials with high thermal conductivity or non-uniform geometries. However, ASTM C177 is more time-consuming and expensive than ASTM C518.
-
ASTM C1363 (Standard Test Method for Thermal Performance of Building Materials and Envelope Assemblies by Means of a Hot Box Apparatus): This method is used to measure the thermal performance of complete wall or roof assemblies, including insulation, framing, and cladding. A hot box simulates the interior of a building, while a cold box simulates the exterior. The heat flow through the assembly is measured, and the overall thermal resistance (R-value) is calculated. ASTM C1363 provides a more realistic assessment of thermal performance than ASTM C518 or ASTM C177, but it is also more complex and expensive.
It is important to note that the R-values obtained from these test methods are typically measured under ideal laboratory conditions, which may not accurately reflect real-world performance. Factors such as moisture content, air infiltration, and thermal bridging can significantly reduce the effective R-value of insulation in buildings.
4. Material-Specific Variations in R-value and Performance
The R-value of an insulation material is primarily determined by its composition and structure. Different materials exhibit a wide range of R-values per inch (or per unit thickness), reflecting differences in their thermal conductivity and resistance to convective and radiative heat transfer.
-
Fiberglass: Fiberglass insulation is a widely used and cost-effective option. It typically has an R-value of around 2.2 to 3.7 per inch. Its performance depends on the density and fiber diameter. Higher density fiberglass generally has a higher R-value but can also be more expensive. The interconnected air spaces within the fiberglass matrix are key to its insulation properties.
-
Mineral Wool (Rock Wool and Slag Wool): Mineral wool offers similar thermal performance to fiberglass, with R-values ranging from 3.0 to 4.0 per inch. It is also more fire-resistant than fiberglass, making it a popular choice for applications where fire safety is a concern. Mineral wool tends to be denser than fiberglass and may offer better sound insulation properties.
-
Cellulose: Cellulose insulation is made from recycled paper products and has an R-value of around 3.1 to 3.7 per inch. It is often treated with borates to provide fire resistance and insect control. Cellulose insulation is typically installed as loose-fill insulation, which can effectively fill gaps and cavities, reducing air infiltration. It’s relatively dense, so it performs well with respect to convective loops inside of walls.
-
Spray Foam (Polyurethane and Polyisocyanurate): Spray foam insulation offers high R-values, ranging from 3.5 to 7.0 per inch, depending on the type (open-cell or closed-cell). Closed-cell spray foam has a higher R-value and also acts as an air and moisture barrier. Open-cell spray foam is less expensive but has a lower R-value and does not provide a vapor barrier. The long-term thermal performance of spray foam is sensitive to the composition of gases trapped in the bubbles within the foam. As those gases change over time, the R-value can degrade.
-
Rigid Foam Boards (EPS, XPS, and Polyisocyanurate): Rigid foam boards offer high R-values and are often used for insulating walls, roofs, and foundations. EPS has an R-value of around 3.6 to 4.2 per inch, while XPS has an R-value of around 5.0 per inch. Polyisocyanurate boards have the highest R-value, ranging from 6.0 to 8.0 per inch. Like spray foam, the long-term R-value of these products is also sensitive to the aging of the gases trapped within the foam structure.
-
Vacuum Insulation Panels (VIPs): VIPs offer the highest R-value per inch of any commercially available insulation material, ranging from 20 to 30 per inch. They consist of a porous core material encased in an airtight envelope, with a vacuum drawn inside. VIPs are very effective at reducing conductive and convective heat transfer, but they are also expensive and susceptible to damage if the envelope is punctured.
-
Aerogels: Aerogels are highly porous solid materials with extremely low densities. They offer very high R-values, ranging from 8 to 12 per inch, and are also lightweight and fire-resistant. Aerogels are becoming increasingly popular for niche applications, such as insulating building facades and industrial equipment.
The performance of insulation materials can also be affected by factors such as moisture content, aging, and compression. Moisture can significantly reduce the R-value of many insulation materials, while aging can cause some materials to degrade over time. Compression can reduce the thickness of insulation, which also reduces its R-value. It is important to consider these factors when selecting and installing insulation materials.
5. R-value, Climate Zones, and Energy Efficiency
The optimal R-value for a building’s insulation depends on the climate zone in which it is located. Buildings in colder climates require higher R-values to reduce heat loss during the heating season, while buildings in hotter climates require higher R-values to reduce heat gain during the cooling season. Climate zones are typically defined based on average temperature and humidity levels. The International Energy Conservation Code (IECC) provides recommendations for minimum R-values for different climate zones.
In colder climates, high R-values in walls, roofs, and foundations are essential for minimizing heating energy consumption. In addition to insulation, air sealing is also crucial for preventing air leakage, which can significantly reduce the effectiveness of insulation. In some very cold climates, the impact of the R-value of windows and doors also becomes significant. In hotter climates, high R-values in roofs and walls are important for reducing cooling energy consumption. Shading devices, such as overhangs and awnings, can also help to reduce solar heat gain. In mixed climates, where both heating and cooling are required, a balanced approach to insulation and air sealing is needed.
Increasing insulation levels beyond the minimum recommended R-values can often result in significant energy savings, but the relationship between R-value and energy savings is not always linear. As the R-value increases, the incremental energy savings tend to diminish. This is due to the fact that other factors, such as air infiltration, thermal bridging, and window performance, become more important as the insulation level increases. Furthermore, there is a practical limit to the amount of insulation that can be installed in a building. The diminishing returns of increased R-value, coupled with the increased cost of higher R-value materials and installation, make it essential to conduct a cost-benefit analysis to determine the optimal insulation level for a particular building and climate zone. Simulation tools can be helpful in this analysis, but should be calibrated to measured energy performance to give confidence in the projections.
6. Limitations of R-value as a Sole Indicator of Insulation Performance
While R-value is a useful metric for comparing the thermal resistance of different insulation materials, it has several limitations as a sole indicator of insulation performance in buildings.
-
Thermal Bridging: R-value only considers conductive heat transfer through the insulation material itself. It does not account for thermal bridging, which occurs when heat flows through more conductive elements of the building envelope, such as studs, framing members, and metal connectors. Thermal bridges can significantly reduce the effective R-value of a wall or roof assembly. Calculating the effective R-value of a building envelope requires a more sophisticated analysis that considers the geometry and thermal properties of all the components.
-
Air Infiltration: R-value does not account for heat loss or gain due to air infiltration. Air leakage can significantly increase energy consumption, especially in leaky buildings. Air sealing is therefore crucial for maximizing the effectiveness of insulation. An airtight building envelope can reduce air infiltration to near zero, whereas a leaky building can have an effective R-value significantly below what is calculated based on the insulation alone. While R-value measures resistance to heat flow, air infiltration essentially bypasses the thermal resistance of the building envelope.
-
Radiative Heat Transfer: While R-value implicitly accounts for radiative heat transfer within the insulation material, it does not fully capture the effects of radiative heat transfer across air spaces or through transparent materials such as windows. In some cases, radiant barriers can be used to reduce radiative heat transfer, but their effectiveness depends on the orientation of the surface and the temperature difference between the surfaces.
-
Moisture Effects: As noted earlier, R-value is typically measured under dry conditions. However, the presence of moisture can significantly reduce the R-value of many insulation materials. Moisture can increase the thermal conductivity of the material, and it can also lead to condensation, which can further reduce the insulation’s performance. Building codes often require the use of vapor barriers to prevent moisture from entering the insulation.
-
Dynamic Thermal Conditions: R-value is a steady-state measure of thermal resistance. It does not account for the dynamic effects of solar radiation, temperature fluctuations, and occupancy patterns. The thermal mass of building materials can also affect the building’s thermal performance under dynamic conditions. Thermal mass refers to the ability of a material to store heat. Materials with high thermal mass can help to moderate temperature fluctuations and reduce peak heating and cooling loads.
7. Future Developments in Insulation Performance Metrics
Recognizing the limitations of R-value, researchers and industry professionals are exploring alternative metrics and approaches for evaluating insulation performance. These include:
-
Dynamic R-values: Dynamic R-values would account for the dynamic effects of temperature fluctuations, solar radiation, and occupancy patterns. These metrics would provide a more realistic assessment of insulation performance under real-world conditions. Dynamic R-values could be determined using computer simulations or field measurements.
-
Integrated Thermal Performance Indicators: Integrated thermal performance indicators would combine the effects of insulation, air sealing, thermal bridging, and radiative heat transfer into a single metric. These indicators would provide a more holistic assessment of building envelope performance. One example is the whole-building thermal transmittance (U-value), which considers the combined thermal resistance of all the building envelope components, including walls, roofs, windows, and doors. However, the U-value still doesn’t capture air infiltration effects.
-
Advanced Simulation Tools: Advanced simulation tools, such as building energy modeling (BEM) software, can be used to simulate the thermal performance of buildings under various operating conditions. These tools can account for the effects of insulation, air sealing, thermal bridging, radiative heat transfer, and dynamic thermal conditions. BEM software can be used to optimize building designs and to predict energy consumption. These models, however, need to be carefully validated with measured energy consumption to ensure their accuracy.
-
Phase Change Materials (PCMs): PCMs are materials that absorb and release heat as they undergo a phase change (e.g., solid to liquid). PCMs can be integrated into building materials to increase their thermal mass and to improve their thermal performance. PCMs can help to reduce temperature fluctuations and to reduce peak heating and cooling loads. The effective R-value of a wall or roof assembly with PCMs can vary depending on the temperature and the phase change temperature of the PCM.
-
Adaptive Insulation Materials: Adaptive insulation materials are materials whose thermal properties change in response to environmental conditions. For example, some materials become more insulating at higher temperatures, while others become more insulating at lower temperatures. Adaptive insulation materials could potentially improve the thermal performance of buildings by automatically adjusting to changing conditions. Examples of these kind of materials are still in their early research and development phase.
These future developments aim to provide a more accurate and comprehensive assessment of insulation performance, leading to more energy-efficient and sustainable buildings.
8. Conclusion
R-value remains a valuable, yet limited, metric for assessing the thermal resistance of insulation materials. While it provides a useful benchmark for comparing different materials and for complying with building codes, it does not fully capture the complex interactions of heat transfer mechanisms within building envelopes. Factors such as thermal bridging, air infiltration, radiative heat transfer, and moisture effects can significantly reduce the effective R-value of insulation in buildings. To achieve significant energy savings, it is essential to consider these factors in addition to R-value when designing and constructing buildings. Future developments in insulation performance metrics, such as dynamic R-values, integrated thermal performance indicators, and advanced simulation tools, hold the promise of providing a more accurate and comprehensive assessment of insulation performance, leading to more energy-efficient and sustainable buildings. The integration of these advanced metrics, coupled with a holistic approach to building design and construction, will be crucial for achieving ambitious energy efficiency targets and creating a more sustainable built environment.
References
- American Society for Testing and Materials (ASTM). (Various Standards, e.g., C177, C518, C1363).
- International Energy Conservation Code (IECC).
- ASHRAE Handbook – Fundamentals.
- Straube, J., & Burnett, E. (2011). Building science basics: Thermal control. Building Science Press.
- Lstiburek, J. (2008). Understanding air barriers. Building Science Corporation.
- Kosny, J. (2011). Alternatives to R-value as a measure of thermal performance. Oak Ridge National Laboratory.
- Pacheco-Torgal, F., Ivanov, V., Karakurt, C., Çiftçi, M. K., & Granqvist, C. G. (2021). High-performance thermal insulation for buildings: A review. Construction and Building Materials, 268, 121162.
- Jelle, B. P., & Hynd, A. W. (2011). Vacuum insulation panels (VIPs) for building applications: A review and future directions. Energy and Buildings, 43(10), 2577-2603.
- Baetens, R., Jelle, B. P., & Gustavsen, A. (2011). Aerogel insulation for building applications: A review. Energy and Buildings, 43(4), 761-769.
So, R-value is like the building world’s miles per gallon. Good to know, but real-world conditions will always throw a wrench in the works, right? What about the impact of embodied carbon in different insulation materials? Does chasing a higher R-value always justify the upfront environmental cost?
That’s a great point! You’re absolutely right that embodied carbon is a critical consideration. It’s not just about operational energy savings; we need to factor in the environmental footprint of the materials themselves. A lower R-value material with significantly less embodied carbon might be the better choice overall for a truly sustainable build. What strategies do you find most effective in minimizing embodied carbon in your projects?
Editor: FocusNews.Uk
Thank you to our Sponsor Focus 360 Energy
The review mentions dynamic R-values as a future development. Considering the increasing use of smart building technologies, how might real-time data on occupancy and environmental conditions be integrated to optimize insulation performance and dynamically adjust heating/cooling systems?
That’s a fantastic question! Integrating real-time data with dynamic R-values could revolutionize building management. Imagine sensors adjusting insulation properties based on occupancy, weather, and energy prices. This opens exciting possibilities for personalized comfort and significant energy savings. What types of sensors do you think would be most crucial for such a system?
Editor: FocusNews.Uk
Thank you to our Sponsor Focus 360 Energy
Fascinating stuff! All this talk of R-value limitations makes me wonder: are we about to see a renaissance in traditional building techniques that naturally address those sneaky thermal bridges and air infiltration issues that R-value alone misses?
That’s a really insightful question! It’s definitely worth considering whether we’ll see a return to older methods. Techniques like strawbale construction or timber framing inherently manage some of those issues through material properties and design. Perhaps a blend of traditional wisdom and modern materials could be the most effective path forward?
Editor: FocusNews.Uk
Thank you to our Sponsor Focus 360 Energy