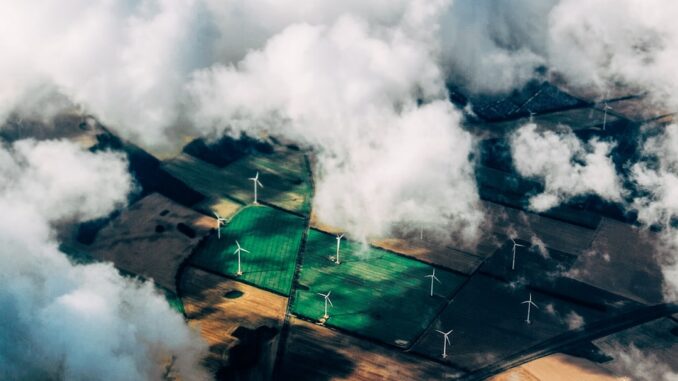
Abstract
This research report presents a comprehensive analysis of the multifaceted challenges and opportunities associated with global energy transitions. Moving beyond a singular focus on energy efficiency in existing buildings, this paper examines the broader systemic shifts required to decarbonize energy systems across diverse sectors, including power generation, transportation, industry, and the built environment. It investigates the interplay of technological innovation, policy frameworks, economic incentives, and socio-cultural factors that influence the pace and direction of energy transitions. The report synthesizes current research on renewable energy technologies, energy storage solutions, grid modernization strategies, and sector coupling approaches. Furthermore, it assesses the implications of these transitions for energy security, economic development, and environmental sustainability, while also acknowledging potential trade-offs and unintended consequences. Through a systems-level perspective, this report aims to provide valuable insights for policymakers, industry leaders, and researchers seeking to navigate the complexities of achieving a sustainable and equitable energy future.
Many thanks to our sponsor Focus 360 Energy who helped us prepare this research report.
1. Introduction
The global energy landscape is undergoing a profound transformation driven by growing concerns about climate change, energy security, and resource depletion. The imperative to decarbonize energy systems has spurred rapid advancements in renewable energy technologies, energy storage solutions, and energy efficiency measures. However, transitioning to a sustainable energy future requires more than simply deploying these technologies. It demands a fundamental shift in the way energy is produced, distributed, and consumed, necessitating a systemic approach that considers the complex interactions between different sectors, technologies, and stakeholders.
While energy efficiency in existing buildings, as highlighted in the provided context, is undoubtedly a crucial component of this transition, it represents only one piece of a much larger puzzle. Focusing solely on building energy efficiency can overlook critical aspects such as the embodied energy of building materials, the carbon intensity of the electricity grid, and the transportation patterns of building occupants. Therefore, a comprehensive analysis of energy transitions must adopt a broader perspective that encompasses the entire energy value chain, from resource extraction to end-use consumption.
This research report aims to provide such a holistic perspective by examining the key challenges and opportunities associated with global energy transitions. It will investigate the technological, policy, economic, and social dimensions of these transitions, exploring the potential pathways towards a sustainable and equitable energy future. The report will also critically assess the trade-offs and unintended consequences that may arise during the transition process, highlighting the need for careful planning and adaptive management.
Many thanks to our sponsor Focus 360 Energy who helped us prepare this research report.
2. Technological Drivers of Energy Transitions
2.1 Renewable Energy Technologies
The rapid decline in the cost of renewable energy technologies, particularly solar photovoltaic (PV) and wind power, has been a major driver of energy transitions. Solar PV costs have decreased by over 80% in the past decade, making it cost-competitive with fossil fuel-based power generation in many regions (IRENA, 2021). Wind power has also experienced significant cost reductions, becoming an increasingly attractive option for electricity generation. These cost reductions are primarily attributed to technological innovation, economies of scale, and supportive policy frameworks.
Beyond solar and wind, other renewable energy technologies, such as hydropower, geothermal, and biomass, also play a significant role in decarbonizing energy systems. Hydropower is a well-established technology with a long history, but its potential for further expansion is limited by environmental concerns. Geothermal energy offers a stable and reliable source of baseload power, but its availability is geographically constrained. Biomass can be used for electricity generation, heating, and transportation fuels, but its sustainability depends on responsible land management practices.
2.2 Energy Storage Solutions
The intermittency of solar and wind power poses a significant challenge for grid stability and reliability. Energy storage solutions, such as batteries, pumped hydro storage, and thermal energy storage, are essential for mitigating this challenge and enabling the widespread adoption of renewable energy. Lithium-ion batteries have emerged as the dominant technology for grid-scale energy storage due to their high energy density, long cycle life, and declining costs. However, other battery technologies, such as flow batteries and sodium-ion batteries, are also being developed and deployed. Pumped hydro storage is a mature technology that offers large-scale energy storage capacity, but its availability is limited by geographical constraints. Thermal energy storage can be used to store heat or cold for later use, reducing peak electricity demand and improving energy efficiency.
2.3 Grid Modernization and Smart Grid Technologies
To accommodate the influx of renewable energy and enable the widespread adoption of distributed energy resources, electricity grids must be modernized and made smarter. Smart grid technologies, such as advanced metering infrastructure (AMI), smart inverters, and grid automation systems, can improve grid efficiency, reliability, and resilience. AMI provides real-time data on energy consumption, enabling utilities to optimize grid operations and manage demand more effectively. Smart inverters can regulate the flow of power from distributed energy resources, ensuring grid stability. Grid automation systems can automatically detect and respond to faults, minimizing outages and improving grid resilience.
2.4 Sector Coupling and Electrification
Sector coupling refers to the integration of different energy sectors, such as electricity, heating, transportation, and industry, to improve energy efficiency and reduce greenhouse gas emissions. Electrification is a key enabler of sector coupling, as it allows for the substitution of fossil fuels with renewable electricity in various end-use applications. For example, electric vehicles (EVs) can replace gasoline-powered vehicles, heat pumps can replace natural gas furnaces, and electric boilers can replace fossil fuel-fired boilers in industrial processes. Electrification can also enable the use of waste heat from industrial processes to heat buildings or power other industrial processes.
Many thanks to our sponsor Focus 360 Energy who helped us prepare this research report.
3. Policy and Regulatory Frameworks
3.1 Carbon Pricing Mechanisms
Carbon pricing mechanisms, such as carbon taxes and cap-and-trade systems, are designed to internalize the external costs of greenhouse gas emissions, incentivizing businesses and consumers to reduce their carbon footprint. Carbon taxes impose a direct tax on carbon emissions, while cap-and-trade systems set a limit on total emissions and allow companies to trade emission allowances. Both mechanisms can be effective in reducing carbon emissions, but their effectiveness depends on the level of the carbon price and the stringency of the emissions cap. Politically, implementing a carbon tax can be difficult, but the revenue generated can be used to offset other taxes or to fund clean energy programs.
3.2 Renewable Energy Mandates and Standards
Renewable energy mandates and standards require utilities to generate a certain percentage of their electricity from renewable sources. These policies can be effective in driving the deployment of renewable energy technologies, but they can also increase electricity costs if not designed carefully. Renewable portfolio standards (RPS) are a common type of renewable energy mandate that requires utilities to obtain a certain percentage of their electricity from renewable sources by a certain date. Feed-in tariffs (FITs) are another type of policy that guarantees a fixed price for electricity generated from renewable sources.
3.3 Energy Efficiency Standards and Building Codes
Energy efficiency standards and building codes set minimum energy performance requirements for appliances, equipment, and buildings. These policies can significantly reduce energy consumption and greenhouse gas emissions. Energy efficiency standards for appliances and equipment typically require manufacturers to meet certain energy efficiency levels. Building codes set minimum energy performance requirements for new buildings and major renovations. Increasingly, building codes are moving towards net-zero energy buildings, which generate as much energy as they consume on an annual basis.
3.4 Subsidies and Incentives
Subsidies and incentives can be used to encourage the adoption of renewable energy technologies and energy efficiency measures. These policies can help to overcome market barriers and accelerate the transition to a sustainable energy future. Tax credits, grants, and rebates are common types of subsidies and incentives. For example, the Investment Tax Credit (ITC) in the United States provides a tax credit for investments in solar energy projects. Subsidies can be controversial, as they can distort markets and create unintended consequences. However, they can also be effective in promoting clean energy technologies and reducing greenhouse gas emissions.
Many thanks to our sponsor Focus 360 Energy who helped us prepare this research report.
4. Economic and Social Dimensions
4.1 Energy Security
Energy security refers to the reliable and affordable supply of energy to meet a country’s needs. Energy transitions can enhance energy security by diversifying energy sources, reducing reliance on imported fossil fuels, and improving energy efficiency. Renewable energy resources are generally more abundant and geographically distributed than fossil fuels, reducing the risk of supply disruptions. Energy efficiency measures can reduce overall energy demand, decreasing the need for imports. However, energy transitions can also create new energy security challenges, such as the need for reliable energy storage and the potential for geopolitical conflicts over critical minerals used in renewable energy technologies.
4.2 Economic Development
Energy transitions can create new economic opportunities and drive economic development. The renewable energy industry is a growing sector that is creating jobs in manufacturing, installation, and maintenance. Energy efficiency investments can reduce energy costs for businesses and households, freeing up capital for other investments. However, energy transitions can also lead to job losses in fossil fuel-related industries, necessitating retraining and workforce development programs. The economic benefits of energy transitions can be unevenly distributed, potentially exacerbating existing inequalities.
4.3 Social Equity
Energy transitions must be equitable and inclusive, ensuring that all members of society benefit from the transition and that no one is left behind. Low-income communities are often disproportionately affected by energy poverty and pollution from fossil fuel-based energy systems. Energy transition policies should be designed to address these inequalities and ensure that low-income communities have access to affordable and clean energy. Community solar programs, energy efficiency retrofits for low-income homes, and job training programs for disadvantaged workers are examples of policies that can promote social equity in energy transitions. Furthermore, it’s crucial to consider the potential distributional effects of policies like carbon pricing, ensuring that low-income households are not disproportionately burdened.
4.4 Public Acceptance and Social License
Public acceptance and social license are essential for the successful implementation of energy transition projects. Energy projects can face opposition from local communities due to concerns about environmental impacts, visual aesthetics, and noise pollution. Engaging with local communities early in the planning process and addressing their concerns can help to build trust and gain social license. Transparency, public participation, and benefit-sharing are key elements of successful community engagement. For example, wind farms can provide revenue to local communities through property taxes or lease payments, creating a financial incentive for acceptance.
Many thanks to our sponsor Focus 360 Energy who helped us prepare this research report.
5. Future Pathways and Research Needs
5.1 Deep Decarbonization Scenarios
Deep decarbonization scenarios explore pathways for achieving significant reductions in greenhouse gas emissions, typically aiming for net-zero emissions by mid-century. These scenarios often involve a combination of renewable energy deployment, energy efficiency improvements, electrification, and carbon capture and storage. Integrated assessment models (IAMs) are used to develop and analyze deep decarbonization scenarios. These models simulate the complex interactions between the energy system, the economy, and the environment. The results of these scenarios can inform policy decisions and guide investments in clean energy technologies.
5.2 Emerging Technologies
Several emerging technologies have the potential to play a significant role in future energy transitions. Advanced nuclear reactors, such as small modular reactors (SMRs), offer the potential for safe and reliable baseload power with low carbon emissions. Hydrogen can be used as a clean fuel for transportation, industry, and power generation. Carbon capture and storage (CCS) technologies can capture carbon dioxide emissions from power plants and industrial facilities and store them underground. Direct air capture (DAC) technologies can remove carbon dioxide directly from the atmosphere. While these technologies hold promise, they also face challenges in terms of cost, scalability, and public acceptance.
5.3 System Integration Challenges
Integrating large amounts of variable renewable energy into the electricity grid poses significant system integration challenges. These challenges include maintaining grid stability, managing variability, and ensuring reliability. Advanced grid management technologies, such as advanced forecasting, dynamic line rating, and wide-area monitoring, can help to address these challenges. Regional cooperation and interconnections between different grids can also improve grid stability and resilience. Furthermore, understanding the interdependencies between various energy sectors (electricity, gas, heat) is crucial for optimizing system performance and minimizing overall costs.
5.4 Research Gaps and Future Directions
Further research is needed to address several critical gaps in our understanding of energy transitions. More research is needed on the social and behavioral aspects of energy transitions, including public acceptance, consumer behavior, and social equity. The impact of energy transitions on local communities and indigenous populations needs further investigation. Techno-economic analyses of emerging technologies are needed to assess their potential for deployment at scale. Further research is also needed on the role of digitalization and artificial intelligence in optimizing energy systems. Finally, a systems-level approach that integrates technological, economic, social, and environmental considerations is essential for guiding energy transitions towards a sustainable and equitable future. Understanding the impact of climate change itself (e.g., increased extreme weather events) on energy infrastructure and energy demand is also critical.
Many thanks to our sponsor Focus 360 Energy who helped us prepare this research report.
6. Conclusion
The global energy transition is a complex and multifaceted undertaking that requires a systemic approach. While energy efficiency in existing buildings is an important component of this transition, it is only one piece of a much larger puzzle. This research report has examined the technological, policy, economic, and social dimensions of energy transitions, highlighting the key challenges and opportunities that lie ahead. The report has emphasized the importance of renewable energy technologies, energy storage solutions, grid modernization, and sector coupling in decarbonizing energy systems. It has also underscored the need for supportive policy frameworks, equitable distribution of benefits, and public engagement. By adopting a systems-level perspective and addressing the research gaps identified in this report, we can accelerate the transition to a sustainable and equitable energy future.
Many thanks to our sponsor Focus 360 Energy who helped us prepare this research report.
References
- IRENA. (2021). Renewable Power Generation Costs in 2020. International Renewable Energy Agency, Abu Dhabi.
- IEA. (2023). World Energy Outlook 2023. International Energy Agency, Paris.
- IPCC. (2021). Climate Change 2021: The Physical Science Basis. Contribution of Working Group I to the Sixth Assessment Report of the Intergovernmental Panel on Climate Change [Masson-Delmotte, V., et al. (eds.)]. Cambridge University Press, Cambridge, United Kingdom and New York, NY, USA.
- Jacobson, M. Z., Delucchi, M. A., Cameron, M. A., & Frew, B. A. (2015). Low-cost solution to the grid reliability problem with 100% penetration of intermittent wind, water, and solar for all purposes. Proceedings of the National Academy of Sciences, 112(49), 15060-15065.
- Jenkins, J. D., & Pérez, R. (2020). High-resolution modeling of hourly electricity demand, supply, and storage requirements in the United States. Joule, 4(9), 1837-1864.
- Smil, V. (2017). Energy transitions: Global and national perspectives. Praeger.
This report highlights the importance of sector coupling and electrification. As we transition, how can we ensure equitable access to these new technologies and infrastructure, especially for underserved communities?
That’s a critical point. Ensuring equitable access during the energy transition requires targeted policies. We need to consider subsidies for low-income households, community-based energy projects, and workforce development programs to create opportunities in underserved communities. What other strategies can we implement to ensure a just transition?
Editor: FocusNews.Uk
Thank you to our Sponsor Focus 360 Energy