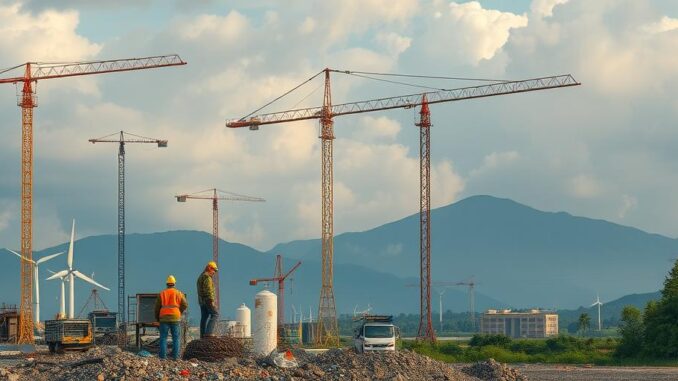
Abstract
Carbon, the cornerstone of organic chemistry and a ubiquitous element in our environment, plays a pivotal role in a vast array of natural and anthropogenic processes. This research report provides a comprehensive overview of carbon, spanning its fundamental atomic structure and diverse allotropes to its complex biogeochemical cycles and its pervasive influence on climate change. We explore the crucial distinction between embodied and operational carbon, particularly within the context of the construction industry. We examine various carbon capture and sequestration technologies, assess the effectiveness of current carbon policies, and discuss the multifaceted challenges and opportunities associated with transitioning to a carbon-neutral future. The report also delves into the global context of carbon emissions, highlighting regional disparities and examining best practices from around the world, with a particular emphasis on innovations in sustainable construction materials and methodologies.
Many thanks to our sponsor Focus 360 Energy who helped us prepare this research report.
1. Introduction: Carbon’s Ubiquity and Significance
Carbon (C), with an atomic number of 6, occupies a unique position in the periodic table. Its ability to form four covalent bonds enables the creation of an unparalleled diversity of molecules, making it the foundation of all known life. Beyond its biological importance, carbon is a key component of numerous geological formations, including diamonds, graphite, and fossil fuels. The carbon cycle, a complex series of processes involving the exchange of carbon between the atmosphere, oceans, land, and living organisms, is fundamental to maintaining Earth’s climate and supporting life.
The rapid increase in atmospheric carbon dioxide (CO2) concentration since the Industrial Revolution, primarily due to the combustion of fossil fuels, has resulted in significant climate change impacts, including rising global temperatures, sea-level rise, and more frequent extreme weather events. Addressing this challenge requires a comprehensive understanding of carbon’s sources, sinks, and transformations, as well as the development and implementation of effective mitigation strategies.
This report aims to provide a detailed analysis of carbon’s role in both natural and anthropogenic systems, with a particular focus on its impact on climate change and strategies for mitigating its effects. The report will address the following key areas:
- Fundamental properties of carbon and its various allotropes.
- The carbon cycle and its key processes.
- Anthropogenic carbon emissions and their impact on the climate.
- Carbon capture and sequestration technologies.
- Carbon policies and their effectiveness.
- The role of the construction industry in carbon emissions, focusing on embodied and operational carbon.
- Innovations in sustainable construction materials and methodologies.
- The global context of carbon emissions and best practices from around the world.
Many thanks to our sponsor Focus 360 Energy who helped us prepare this research report.
2. Carbon’s Atomic Structure and Allotropes
The electronic configuration of carbon (1s2 2s2 2p2) allows it to form four covalent bonds, leading to a vast array of organic molecules. This tetravalency is the key to carbon’s unique ability to form complex structures and is the basis of organic chemistry.
Carbon exists in several allotropic forms, each with distinct physical and chemical properties:
- Diamond: A highly ordered, three-dimensional crystal lattice where each carbon atom is bonded to four other carbon atoms in a tetrahedral arrangement. This structure gives diamond its exceptional hardness and high refractive index. Diamond is widely used in industrial cutting tools and as a gemstone.
- Graphite: Consists of layers of carbon atoms arranged in hexagonal rings. Within each layer, carbon atoms are strongly bonded, but the layers are held together by weaker van der Waals forces. This structure allows graphite to be a good electrical conductor and a lubricant.
- Fullerenes: Spherical or ellipsoidal molecules composed of carbon atoms arranged in pentagonal and hexagonal rings. The most well-known fullerene is C60 (buckminsterfullerene), a spherical molecule consisting of 60 carbon atoms. Fullerenes have potential applications in nanotechnology, drug delivery, and materials science.
- Carbon Nanotubes: Cylindrical structures consisting of rolled-up sheets of graphene (a single layer of graphite). Carbon nanotubes possess exceptional strength, electrical conductivity, and thermal conductivity, making them promising materials for a wide range of applications, including electronics, composites, and energy storage.
- Graphene: A single layer of carbon atoms arranged in a hexagonal lattice. Graphene is the basic building block of graphite, carbon nanotubes, and fullerenes. It exhibits exceptional electrical conductivity, mechanical strength, and thermal conductivity, making it a promising material for electronics, sensors, and composites.
- Amorphous Carbon: Lacks long-range order and is typically formed by the rapid cooling of molten carbon or by the decomposition of carbon-containing compounds. Amorphous carbon is used in coatings, thin films, and as a component of various materials.
The properties of these allotropes are directly related to their atomic structure and bonding. The diverse range of properties exhibited by carbon allotropes makes carbon a versatile material with applications in a wide range of fields.
Many thanks to our sponsor Focus 360 Energy who helped us prepare this research report.
3. The Carbon Cycle: A Complex System of Exchanges
The carbon cycle is a biogeochemical cycle that describes the movement of carbon atoms through various reservoirs, including the atmosphere, oceans, land, and living organisms. The cycle involves several key processes:
- Photosynthesis: The process by which plants and other photosynthetic organisms convert carbon dioxide (CO2) from the atmosphere into organic compounds using sunlight as an energy source. Photosynthesis is a major carbon sink, removing CO2 from the atmosphere and storing it in plant biomass.
- Respiration: The process by which living organisms break down organic compounds to release energy, producing CO2 as a byproduct. Respiration returns CO2 to the atmosphere and oceans.
- Decomposition: The breakdown of dead organic matter by decomposers (bacteria and fungi), releasing CO2 and other nutrients back into the environment. Decomposition plays a crucial role in recycling carbon and other elements.
- Ocean Exchange: The oceans absorb CO2 from the atmosphere through direct dissolution and through biological processes, such as photosynthesis by phytoplankton. The oceans also release CO2 back into the atmosphere through respiration and decomposition.
- Volcanic Activity: Volcanoes release CO2 from the Earth’s interior into the atmosphere. While volcanic emissions are a natural source of CO2, they are relatively small compared to anthropogenic emissions.
- Sedimentation and Burial: Over long periods, organic matter can be buried in sediments and transformed into fossil fuels (coal, oil, and natural gas). This process removes carbon from the active cycle and stores it in geological reservoirs.
- Combustion: The burning of fossil fuels and biomass releases CO2 into the atmosphere. Combustion is a major anthropogenic source of CO2.
The carbon cycle is a dynamic system, and the fluxes of carbon between reservoirs can vary over time due to natural and anthropogenic factors. Human activities, particularly the burning of fossil fuels and deforestation, have significantly altered the carbon cycle, leading to a rapid increase in atmospheric CO2 concentration.
Many thanks to our sponsor Focus 360 Energy who helped us prepare this research report.
4. Anthropogenic Carbon Emissions and Climate Change
The Industrial Revolution marked a turning point in the carbon cycle, as the widespread use of fossil fuels for energy production led to a dramatic increase in anthropogenic carbon emissions. The burning of coal, oil, and natural gas releases CO2 into the atmosphere, increasing its concentration and contributing to the greenhouse effect.
The greenhouse effect is a natural process that warms the Earth’s surface. Greenhouse gases, such as CO2, methane (CH4), and nitrous oxide (N2O), absorb infrared radiation emitted by the Earth’s surface, trapping heat in the atmosphere. Without the greenhouse effect, the Earth would be too cold to support life.
However, the increased concentration of greenhouse gases in the atmosphere due to anthropogenic emissions has enhanced the greenhouse effect, leading to global warming. Global warming is causing a wide range of climate change impacts, including:
- Rising Global Temperatures: The Earth’s average temperature has increased significantly since the Industrial Revolution, and further warming is expected in the future.
- Sea-Level Rise: Melting glaciers and ice sheets, as well as thermal expansion of seawater, are causing sea levels to rise, threatening coastal communities and ecosystems.
- Extreme Weather Events: Climate change is contributing to more frequent and intense extreme weather events, such as heat waves, droughts, floods, and hurricanes.
- Ocean Acidification: The absorption of CO2 by the oceans is causing them to become more acidic, threatening marine life, particularly shellfish and coral reefs.
Reducing anthropogenic carbon emissions is crucial to mitigating climate change. This requires a transition to a low-carbon economy based on renewable energy sources, energy efficiency, and sustainable land management practices.
Many thanks to our sponsor Focus 360 Energy who helped us prepare this research report.
5. Carbon Capture and Sequestration Technologies
Carbon capture and sequestration (CCS) technologies aim to capture CO2 from industrial sources and store it underground, preventing it from entering the atmosphere. CCS technologies can be applied to power plants, industrial facilities, and other sources of CO2 emissions.
There are several different CCS technologies:
- Post-Combustion Capture: CO2 is captured from the flue gas after combustion. This is the most widely used CCS technology.
- Pre-Combustion Capture: Fuel is partially oxidized before combustion, producing a mixture of CO2 and hydrogen (H2). The CO2 is then separated from the H2 before combustion.
- Oxy-Fuel Combustion: Fuel is burned in pure oxygen, producing a flue gas consisting primarily of CO2 and water vapor. The CO2 can then be easily captured.
Once captured, CO2 is transported to a storage site, where it is injected into deep geological formations, such as depleted oil and gas reservoirs or saline aquifers. The CO2 is stored underground for long periods, preventing it from entering the atmosphere.
CCS technologies have the potential to significantly reduce carbon emissions from industrial sources. However, CCS is still a relatively expensive technology, and its widespread deployment requires further research, development, and demonstration. The long-term safety and environmental impacts of CO2 storage also need to be carefully assessed.
Many thanks to our sponsor Focus 360 Energy who helped us prepare this research report.
6. Carbon Policies and their Effectiveness
Various carbon policies have been implemented around the world to reduce carbon emissions. These policies can be broadly classified into two categories:
- Carbon Pricing: Carbon pricing mechanisms put a price on carbon emissions, either through a carbon tax or a cap-and-trade system. A carbon tax directly taxes carbon emissions, while a cap-and-trade system sets a limit (cap) on total emissions and allows companies to trade emission allowances (permits). Carbon pricing incentivizes companies to reduce their emissions by making it more expensive to pollute.
- Regulations and Standards: Regulations and standards set specific requirements for carbon emissions or energy efficiency. For example, fuel efficiency standards for vehicles or building codes that require energy-efficient construction.
The effectiveness of carbon policies depends on several factors, including the level of the carbon price, the stringency of regulations, and the enforcement of policies. Carbon pricing is generally considered to be the most economically efficient way to reduce carbon emissions, as it allows companies to choose the most cost-effective way to reduce their emissions. However, carbon pricing can be politically challenging to implement, as it may face opposition from industries that would be affected by the price on carbon.
Regulations and standards can be effective in achieving specific emission reduction targets, but they may be less flexible and less cost-effective than carbon pricing. A combination of carbon pricing and regulations is often used to achieve emission reduction goals.
The effectiveness of carbon policies also depends on the context in which they are implemented. Policies that are effective in one country may not be effective in another country due to differences in economic conditions, political systems, and cultural norms.
Many thanks to our sponsor Focus 360 Energy who helped us prepare this research report.
7. The Role of the Construction Industry: Embodied and Operational Carbon
The construction industry is a significant contributor to global carbon emissions, accounting for a substantial portion of both embodied and operational carbon. Reducing carbon emissions in the construction sector is crucial for achieving global climate goals.
Embodied Carbon: Refers to the total greenhouse gas emissions associated with the extraction, manufacturing, transportation, assembly, and end-of-life stages of building materials and components. This includes emissions from:
- Raw Material Extraction: Mining and quarrying of raw materials such as cement, steel, aluminum, and aggregates.
- Manufacturing: Production of building materials, including cement, steel, aluminum, glass, and plastics.
- Transportation: Shipping and trucking of materials from manufacturing sites to construction sites.
- Construction: On-site assembly and installation of building materials.
- Demolition and Disposal: End-of-life processes, including demolition, transportation, and disposal of building materials.
Operational Carbon: Refers to the greenhouse gas emissions associated with the energy consumption of a building during its operational lifetime. This includes emissions from:
- Heating, Ventilation, and Air Conditioning (HVAC): Energy used for heating, cooling, and ventilation.
- Lighting: Energy used for lighting the building.
- Appliances and Equipment: Energy used by appliances, computers, and other equipment.
- Water Heating: Energy used for heating water.
Reducing embodied carbon requires using low-carbon building materials, optimizing material use, and designing for deconstruction and reuse. Reducing operational carbon requires improving energy efficiency, using renewable energy sources, and designing buildings that minimize energy consumption.
Many thanks to our sponsor Focus 360 Energy who helped us prepare this research report.
8. Innovations in Sustainable Construction Materials and Methodologies
Several innovations are emerging in sustainable construction materials and methodologies to reduce both embodied and operational carbon:
- Low-Carbon Concrete: Concrete is a major source of embodied carbon, due to the high energy intensity of cement production. Low-carbon concrete mixes use alternative cementitious materials, such as fly ash, slag, and geopolymers, to reduce the amount of cement required.
- Sustainable Timber: Timber is a renewable building material that can sequester carbon from the atmosphere. Sustainable timber is sourced from sustainably managed forests and is treated to prevent decay and insect infestation. Mass timber products, such as cross-laminated timber (CLT), are increasingly being used in tall building construction.
- Recycled and Reclaimed Materials: Using recycled and reclaimed materials, such as recycled steel, reclaimed wood, and recycled plastic, reduces the need for new materials and minimizes embodied carbon.
- Modular Construction: Modular construction involves prefabricating building components in a factory and then assembling them on-site. Modular construction can reduce waste, improve construction speed, and reduce embodied carbon.
- 3D Printing: 3D printing of building components is an emerging technology that can reduce waste, improve design flexibility, and potentially reduce embodied carbon. 3D printing can be used to create complex shapes and structures using materials such as concrete, polymers, and composites.
- Passive Design Strategies: Passive design strategies, such as optimizing building orientation, using natural ventilation, and incorporating shading devices, can reduce the need for mechanical heating and cooling, reducing operational carbon.
- Building Information Modeling (BIM): BIM is a digital representation of a building that can be used to optimize design, construction, and operation. BIM can be used to analyze the carbon footprint of a building and identify opportunities to reduce embodied and operational carbon.
Many thanks to our sponsor Focus 360 Energy who helped us prepare this research report.
9. Global Context and Best Practices
Carbon emissions from the construction industry vary significantly across different countries and regions, depending on factors such as building codes, construction practices, and energy sources. Several countries have implemented policies and initiatives to promote low-carbon construction.
- Europe: The European Union (EU) has implemented a range of policies to reduce carbon emissions from buildings, including the Energy Performance of Buildings Directive (EPBD) and the Construction Products Regulation (CPR). The EPBD sets minimum energy performance standards for new and renovated buildings, while the CPR regulates the environmental performance of construction products.
- North America: The United States and Canada have implemented various policies to promote energy efficiency in buildings, including building codes, tax incentives, and energy efficiency programs. The U.S. Green Building Council’s LEED (Leadership in Energy and Environmental Design) rating system is widely used to certify green buildings.
- Asia: Several countries in Asia, including China, Japan, and South Korea, have implemented policies to promote sustainable construction. China has implemented a green building rating system and has set targets for reducing energy consumption in buildings. Japan has implemented a building energy efficiency standard and has provided subsidies for energy-efficient buildings.
Best practices for reducing carbon emissions in construction include:
- Life Cycle Assessment (LCA): Conducting a life cycle assessment of a building to identify the major sources of embodied and operational carbon.
- Design for Disassembly (DfD): Designing buildings that can be easily disassembled and reused at the end of their life.
- Material Selection: Selecting low-carbon building materials and minimizing material waste.
- Energy Efficiency: Designing buildings that are energy-efficient and using renewable energy sources.
- Collaboration: Collaboration between architects, engineers, contractors, and policymakers to promote sustainable construction practices.
Many thanks to our sponsor Focus 360 Energy who helped us prepare this research report.
10. Challenges and Opportunities
Transitioning to a carbon-neutral future presents both significant challenges and opportunities. Some of the key challenges include:
- Technological Barriers: Developing and deploying cost-effective carbon capture and sequestration technologies.
- Policy Barriers: Implementing effective carbon policies that are politically feasible and economically sound.
- Economic Barriers: Overcoming the high upfront costs of low-carbon technologies and materials.
- Social Barriers: Changing consumer behavior and overcoming resistance to new technologies.
Despite these challenges, there are also significant opportunities:
- Economic Growth: Investing in low-carbon technologies can create new jobs and stimulate economic growth.
- Improved Health: Reducing air pollution from fossil fuels can improve public health.
- Energy Security: Transitioning to renewable energy sources can reduce dependence on imported fossil fuels.
- Environmental Benefits: Reducing carbon emissions can help to protect ecosystems and biodiversity.
Many thanks to our sponsor Focus 360 Energy who helped us prepare this research report.
11. Conclusion
Carbon is a fundamental element that plays a critical role in both natural and anthropogenic systems. Addressing the challenge of climate change requires a comprehensive understanding of carbon’s sources, sinks, and transformations, as well as the development and implementation of effective mitigation strategies. The construction industry has a significant role to play in reducing carbon emissions, through the adoption of sustainable materials, energy-efficient designs, and innovative construction methodologies. By embracing these changes, the construction industry can contribute to a more sustainable and resilient future.
Many thanks to our sponsor Focus 360 Energy who helped us prepare this research report.
References
- Intergovernmental Panel on Climate Change (IPCC). (2021). Climate Change 2021: The Physical Science Basis. Contribution of Working Group I to the Sixth Assessment Report of the Intergovernmental Panel on Climate Change [Masson-Delmotte, V., et al. (eds.)]. Cambridge University Press.
- Ritchie, H., & Roser, M. (2020). CO₂ and Greenhouse Gas Emissions. Our World in Data. Retrieved from https://ourworldindata.org/co2-and-other-greenhouse-gas-emissions
- World Green Building Council. (2019). Bringing Embodied Carbon Upfront. Retrieved from https://www.worldgbc.org/bringing-embodied-carbon-upfront
- United Nations Environment Programme (UNEP). (2021). Global Status Report for Buildings and Construction 2021. Retrieved from https://www.unep.org/resources/report/2021-global-status-report-buildings-and-construction
- European Commission. (n.d.). Energy Performance of Buildings Directive. Retrieved from https://energy.ec.europa.eu/topics/energy-efficiency/energy-performance-buildings_en
- Hammond, G., & Jones, C. (2011). Inventory of Carbon & Energy (ICE) database. University of Bath. https://doi.org/10.13140/RG.2.1.3008.6649
- Pomponi, F., & Moncaster, A. (2017). Embodied carbon for buildings: A review. Renewable and Sustainable Energy Reviews, 60, 112-130.
- Giesekam, J., Barrett, M., & Taylor, J. (2016). Embodied carbon benchmarks for common building materials: A review. Journal of Cleaner Production, 112, 2353-2363.
- Birgisdottir, H., et al. (2017). Integration of Life Cycle Assessment in Building Design and Construction. Buildings, 7(3), 63.
- Allin, R., et al. (2020). Carbon Metrics for the Whole Building Lifecycle. RICS. Retrieved from https://www.rics.org/globalassets/rics-website/media/upholding-professional-standards/sector-standards/building-surveying/carbon-metrics-for-the-whole-building-lifecycle-1st-edition.pdf
The discussion of embodied vs. operational carbon in construction is critical. Exploring innovative financing models could accelerate the adoption of sustainable materials, addressing the upfront cost barrier you mentioned. Perhaps green bonds or tax incentives could play a larger role.
Thanks for your comment! Green bonds and tax incentives are definitely worth exploring. We need to think creatively about how to lower the initial investment hurdle for sustainable materials. Perhaps public-private partnerships could also help drive adoption and innovation in this space. What are your thoughts?
Editor: FocusNews.Uk
Thank you to our Sponsor Focus 360 Energy
Diamonds *are* forever, but perhaps amorphous carbon is the unsung hero? Think of all those sleek coatings and thin films quietly doing their thing. What innovative applications do you see emerging for it in the future?