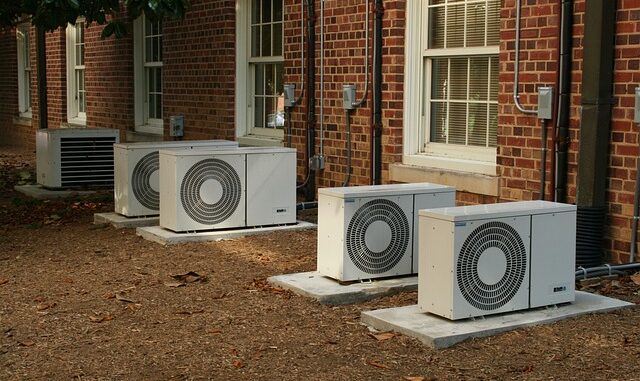
Abstract
Heating, ventilation, and air conditioning (HVAC) systems represent a significant portion of global energy consumption in buildings. This research report provides a comprehensive overview of advanced HVAC technologies, exploring their potential to enhance energy efficiency, improve indoor air quality (IAQ), and facilitate grid integration. The report examines various HVAC system types, including variable refrigerant flow (VRF) systems, geothermal heat pumps, radiant heating and cooling systems, and desiccant-based dehumidification systems. Furthermore, it analyzes advanced control strategies, such as model predictive control (MPC) and artificial intelligence (AI)-driven optimization, for maximizing HVAC system performance. The report also delves into the integration of HVAC systems with renewable energy sources and smart grid technologies, paving the way for more sustainable and resilient building operations. Finally, the report identifies key challenges and opportunities for future research and development in the field of advanced HVAC systems.
Many thanks to our sponsor Focus 360 Energy who helped us prepare this research report.
1. Introduction
The built environment is a major consumer of energy worldwide, with HVAC systems accounting for a substantial portion of this consumption [1]. As global concerns about climate change intensify, reducing energy consumption and carbon emissions from buildings has become a critical priority. Furthermore, maintaining acceptable IAQ is essential for occupant health and productivity [2]. Advanced HVAC systems offer the potential to simultaneously address energy efficiency and IAQ concerns while also enabling greater integration with renewable energy sources and the electricity grid.
Traditional HVAC systems often rely on inefficient technologies and control strategies, resulting in significant energy waste and suboptimal IAQ. In contrast, advanced HVAC systems incorporate innovative technologies and sophisticated controls to optimize system performance, reduce energy consumption, and improve IAQ. These systems can also leverage data analytics and predictive modeling to anticipate future heating and cooling demands, enabling proactive system adjustments that minimize energy use and maximize occupant comfort.
This report aims to provide a comprehensive overview of advanced HVAC systems, exploring their key features, benefits, and challenges. The report will cover a range of topics, including:
- Detailed examination of different types of advanced HVAC systems, such as VRF systems, geothermal heat pumps, radiant heating and cooling systems, and desiccant-based dehumidification systems.
- Analysis of advanced control strategies, including MPC and AI-driven optimization, for enhancing HVAC system performance.
- Discussion of the integration of HVAC systems with renewable energy sources and smart grid technologies.
- Identification of key research and development opportunities in the field of advanced HVAC systems.
Many thanks to our sponsor Focus 360 Energy who helped us prepare this research report.
2. Advanced HVAC System Technologies
2.1 Variable Refrigerant Flow (VRF) Systems
VRF systems are a type of ductless HVAC system that uses refrigerant as the working fluid to provide heating and cooling to multiple zones within a building. These systems offer several advantages over traditional HVAC systems, including: improved energy efficiency, flexible zoning capabilities, and reduced installation costs [3]. VRF systems utilize a variable-speed compressor that adjusts the refrigerant flow rate based on the heating and cooling demands of each zone, minimizing energy waste and providing precise temperature control. The ability to heat some zones while simultaneously cooling others using heat recovery technology offers a substantial energy saving compared to conventional systems. However, correct refrigerant charge is critical for efficient and reliable operation. Leak detection systems are also becoming important.
VRF systems are particularly well-suited for buildings with diverse heating and cooling needs, such as office buildings, hotels, and hospitals. However, the complexity of VRF systems necessitates skilled technicians for installation and maintenance. Furthermore, the environmental impact of refrigerants used in VRF systems is a concern, prompting research into alternative refrigerants with lower global warming potential.
2.2 Geothermal Heat Pumps (GHPs)
GHPs, also known as ground-source heat pumps, utilize the Earth’s relatively constant temperature as a heat source in winter and a heat sink in summer. These systems circulate a fluid through a network of underground pipes, extracting heat from the ground in winter and transferring heat to the ground in summer [4]. GHPs offer several advantages over traditional HVAC systems, including: high energy efficiency, reduced greenhouse gas emissions, and long lifespan. The Co-efficient of Performance (COP) can be significantly higher than air source heat pumps, particularly in regions with extreme temperature swings.
GHPs are suitable for a wide range of building types, from residential homes to commercial buildings. However, the initial installation cost of GHPs can be higher than that of traditional HVAC systems, due to the expense of installing the underground pipe network. Furthermore, the geological characteristics of the site can significantly impact the performance and cost-effectiveness of GHPs. Careful site assessment and system design are crucial for ensuring optimal performance.
2.3 Radiant Heating and Cooling Systems
Radiant heating and cooling systems use water or air to transfer heat directly to or from occupants through radiation and convection. These systems can be integrated into floors, walls, or ceilings, providing a more comfortable and energy-efficient alternative to traditional forced-air systems [5]. Radiant systems provide a more uniform temperature distribution throughout the space, eliminating hot and cold spots and reducing the need for high air velocities. This leads to improved thermal comfort and reduced energy consumption. Moreover, radiant systems can operate at lower temperatures than forced-air systems, making them well-suited for integration with renewable energy sources, such as solar thermal collectors.
While radiant systems offer significant advantages, they also present some challenges. The response time of radiant systems can be slower than that of forced-air systems, requiring careful control strategies to maintain desired temperatures. Furthermore, the installation of radiant systems can be more complex and expensive than that of traditional systems, particularly in retrofit applications. Building construction methods also have a significant impact on system performance.
2.4 Desiccant-Based Dehumidification Systems
Desiccant-based dehumidification systems use a desiccant material to remove moisture from the air. These systems can be particularly effective in humid climates, where high humidity levels can significantly increase cooling loads and reduce IAQ [6]. Desiccant systems can be powered by waste heat or renewable energy sources, such as solar thermal collectors, making them a more sustainable alternative to traditional dehumidification methods. Furthermore, desiccant systems can improve IAQ by removing pollutants and allergens from the air.
Desiccant systems are commonly used in applications such as supermarkets, hospitals, and museums, where precise humidity control is critical. However, the regeneration of the desiccant material requires a heat source, which can be energy-intensive if not properly managed. Advanced desiccant systems incorporate heat recovery and optimized control strategies to minimize energy consumption.
Many thanks to our sponsor Focus 360 Energy who helped us prepare this research report.
3. Advanced Control Strategies
3.1 Model Predictive Control (MPC)
MPC is an advanced control strategy that uses a mathematical model of the HVAC system and the building to predict future performance and optimize control actions. MPC takes into account factors such as weather forecasts, occupancy schedules, and energy prices to determine the optimal control settings for the HVAC system, minimizing energy consumption while maintaining desired comfort levels [7]. MPC can significantly improve the energy efficiency and performance of HVAC systems, particularly in buildings with complex thermal dynamics. It allows the system to proactively adapt to changing conditions and optimize its operation accordingly.
Implementing MPC requires a detailed model of the building and the HVAC system, as well as sophisticated optimization algorithms. The accuracy of the model is crucial for the effectiveness of MPC. Furthermore, the computational complexity of MPC can be a challenge, requiring powerful computing resources. However, advancements in computing technology and model simplification techniques are making MPC more accessible and cost-effective.
3.2 Artificial Intelligence (AI)-Driven Optimization
AI-driven optimization techniques, such as machine learning and neural networks, can be used to analyze large datasets of building and HVAC system performance data to identify patterns and optimize control strategies. AI algorithms can learn from historical data and adapt to changing conditions, continuously improving the performance of the HVAC system [8]. AI-driven optimization can be particularly effective in buildings with complex and dynamic operating conditions. Self-learning algorithms can discover non-intuitive control strategies that outperform traditional methods.
AI-driven optimization requires large amounts of data for training the algorithms. The quality and completeness of the data are crucial for the accuracy and reliability of the AI models. Furthermore, the interpretability of AI models can be a challenge, making it difficult to understand why the algorithms are making certain control decisions. However, explainable AI (XAI) techniques are being developed to address this issue.
Many thanks to our sponsor Focus 360 Energy who helped us prepare this research report.
4. Integration with Renewable Energy Sources and Smart Grid Technologies
4.1 Renewable Energy Integration
Integrating HVAC systems with renewable energy sources, such as solar thermal collectors and photovoltaic (PV) panels, can significantly reduce the reliance on fossil fuels and lower carbon emissions. Solar thermal collectors can provide heat for domestic hot water, space heating, and desiccant dehumidification systems [9]. PV panels can generate electricity to power HVAC equipment, such as heat pumps and chillers. By matching the energy supply with the building’s energy demands, the system can maximize the use of renewable energy and minimize grid reliance.
The integration of HVAC systems with renewable energy sources requires careful system design and control strategies. The intermittent nature of renewable energy sources can pose challenges for maintaining stable and reliable operation. Energy storage technologies, such as thermal energy storage and battery storage, can be used to mitigate these challenges and ensure a continuous supply of energy.
4.2 Smart Grid Integration
Smart grid technologies enable two-way communication between buildings and the electricity grid, allowing HVAC systems to respond to grid signals and adjust their energy consumption accordingly. Demand response programs incentivize building owners to reduce their energy consumption during peak demand periods, helping to stabilize the grid and prevent blackouts [10]. Smart thermostats and building automation systems can automatically adjust HVAC settings in response to grid signals, without compromising occupant comfort.
Smart grid integration requires advanced communication and control infrastructure. Cybersecurity is a critical concern, as buildings become more connected to the grid. Furthermore, the economic benefits of smart grid integration need to be clearly demonstrated to incentivize building owners to participate in demand response programs. Regulatory frameworks are also needed to support the deployment of smart grid technologies and ensure fair access to the grid.
Many thanks to our sponsor Focus 360 Energy who helped us prepare this research report.
5. Challenges and Opportunities
5.1 Challenges
- High Initial Cost: Advanced HVAC systems often have higher initial costs than traditional systems, which can be a barrier to adoption.
- Complexity: Advanced HVAC systems are more complex than traditional systems, requiring specialized knowledge and skills for installation, operation, and maintenance.
- Data Requirements: Advanced control strategies, such as MPC and AI-driven optimization, require large amounts of data for training and operation.
- Cybersecurity: The integration of HVAC systems with smart grids and the internet of things (IoT) raises cybersecurity concerns.
- Refrigerant Regulations: Increasingly strict regulations on refrigerants with high global warming potential are pushing the industry to adopt new and potentially less efficient alternatives.
5.2 Opportunities
- Reduced Energy Consumption: Advanced HVAC systems can significantly reduce energy consumption, leading to lower operating costs and reduced carbon emissions.
- Improved Indoor Air Quality: Advanced HVAC systems can improve IAQ, creating healthier and more comfortable indoor environments.
- Enhanced Grid Integration: Advanced HVAC systems can facilitate grid integration, enabling demand response and the use of renewable energy sources.
- Development of New Technologies: There is a significant opportunity for research and development of new and innovative HVAC technologies, such as advanced heat exchangers, alternative refrigerants, and smart control algorithms.
- Standardization and Interoperability: Developing standardized communication protocols and interoperability standards can facilitate the integration of different HVAC components and systems.
- Life Cycle Cost Analysis: Promoting life cycle cost analysis can demonstrate the long-term economic benefits of advanced HVAC systems.
Many thanks to our sponsor Focus 360 Energy who helped us prepare this research report.
6. Conclusion
Advanced HVAC systems offer significant potential to enhance energy efficiency, improve IAQ, and facilitate grid integration in buildings. While challenges remain, the benefits of these systems are increasingly compelling, driving innovation and adoption. Further research and development are needed to overcome the challenges and unlock the full potential of advanced HVAC systems. Key areas of focus include: reducing initial costs, simplifying system design and operation, developing new and improved technologies, and promoting standardization and interoperability. By addressing these challenges and capitalizing on the opportunities, we can pave the way for a more sustainable and comfortable built environment.
Many thanks to our sponsor Focus 360 Energy who helped us prepare this research report.
References
[1] Pérez-Lombard, L., Ortiz, J., & Pout, C. (2008). A review on buildings energy consumption information. Energy and Buildings, 40(3), 394-398.
[2] Sundell, J. (2004). Indoor air quality and health—A critical review. Indoor Air, 14(S7), 51-58.
[3] Deng, S. M., Burnett, J. W., & Strachan, P. A. (2012). A review of variable refrigerant flow air conditioning systems—Part 1: Modelling and control. Applied Thermal Engineering, 37, 28-42.
[4] Kavanaugh, S. P., & Rafferty, K. (2014). Ground-source heat pumps: Design of geothermal systems for commercial and institutional buildings. ASHRAE.
[5] Olesen, B. W. (2016). A review of radiant heating and cooling systems. REHVA Journal, 53(5), 42-49.
[6] Dai, Y. J., Wang, R. Z., & Zhang, H. F. (2001). Parametric analysis and optimization of a desiccant cooling system. Applied Thermal Engineering, 21(1), 1-15.
[7] Afram, A., & Janabi-Sharifi, F. (2014). Theory and applications of hierarchical model predictive control in building energy management. Energies, 7(3), 2089-2117.
[8] Wang, Z., Hong, T., & Piette, M. A. (2012). Automated commissioning using data analysis and visualization techniques. Energy and Buildings, 48, 100-112.
[9] Duffie, J. A., & Beckman, W. A. (2013). Solar engineering of thermal processes. John Wiley & Sons.
[10] Gellings, C. W. (2009). The smart grid: Enabling energy efficiency and demand response. CRC press.
Fascinating stuff! Makes you wonder if we’ll all be managing our buildings’ energy use with AI while sipping iced tea on Mars one day. Wonder if VRF systems will work in a biodome…