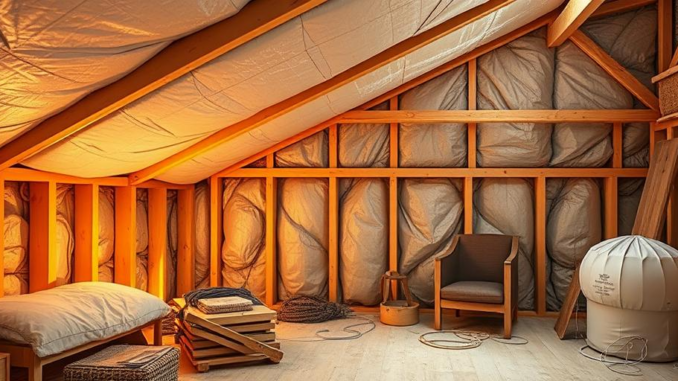
Advanced Insulation Strategies for Sustainable Building Envelopes: A Comprehensive Review
Many thanks to our sponsor Focus 360 Energy who helped us prepare this research report.
Abstract
This research report provides a comprehensive overview of advanced insulation strategies for sustainable building envelopes, addressing the critical role of insulation in enhancing energy efficiency, reducing greenhouse gas emissions, and improving indoor environmental quality. The report delves into the complexities of thermal performance metrics, including R-values, U-factors, and thermal bridging effects, and explores the nuances of various insulation materials, ranging from conventional fiberglass and mineral wool to advanced options like vacuum insulation panels (VIPs), aerogels, and phase change materials (PCMs). Furthermore, the report examines the impact of moisture management on insulation performance and durability, highlighting the importance of vapor retarders and air barriers. Installation techniques, cost-effectiveness analyses, life cycle assessments, and environmental considerations are also addressed, providing a holistic perspective on the selection and implementation of insulation strategies. The report concludes by outlining future trends and research directions in the field of insulation, emphasizing the need for innovative materials, improved modeling tools, and integrated design approaches to achieve high-performance, sustainable building envelopes.
Many thanks to our sponsor Focus 360 Energy who helped us prepare this research report.
1. Introduction
The building sector is a significant consumer of energy, accounting for a substantial portion of global greenhouse gas emissions. A well-insulated building envelope is a fundamental component of sustainable building design, playing a critical role in minimizing energy consumption for heating and cooling. Effective insulation reduces heat transfer through walls, roofs, and floors, thereby maintaining comfortable indoor temperatures and reducing reliance on energy-intensive HVAC systems. This, in turn, leads to lower operating costs for building owners and a reduced environmental footprint.
However, the selection and implementation of insulation strategies are not straightforward. A multitude of factors must be considered, including climate, building type, construction materials, occupancy patterns, budget constraints, and environmental regulations. Furthermore, the performance of insulation is affected by several variables, such as installation quality, moisture content, and thermal bridging. This report aims to provide a comprehensive overview of the various aspects of insulation, covering materials, performance metrics, installation techniques, and environmental considerations. It delves into both conventional and advanced insulation technologies, providing insights into their suitability for different applications and highlighting the latest innovations in the field.
Many thanks to our sponsor Focus 360 Energy who helped us prepare this research report.
2. Thermal Performance Metrics and Principles
2.1 R-Value and U-Factor
The thermal performance of insulation is primarily quantified by its R-value and U-factor. The R-value represents the thermal resistance of a material, indicating its ability to resist heat flow. A higher R-value signifies better insulation performance. Conversely, the U-factor represents the thermal transmittance, indicating the rate of heat flow through a material or assembly. A lower U-factor indicates better insulation performance.
The R-value of a material is determined by its thermal conductivity (k) and its thickness (t): R = t/k. Thermal conductivity is an intrinsic property of the material, while the R-value is dependent on the thickness of the material. It is important to note that the R-value is typically specified for a particular temperature and density. Changes in temperature or density can affect the R-value of the material.
The U-factor, on the other hand, is the inverse of the total thermal resistance of an assembly, including the insulation, framing, sheathing, and air films: U = 1/Rtotal. The U-factor is a more comprehensive measure of the thermal performance of a building envelope component, as it accounts for the contributions of all the materials and air gaps in the assembly.
2.2 Thermal Bridging
Thermal bridging occurs when a highly conductive material penetrates the insulation layer, creating a pathway for heat to flow more easily through the building envelope. Common examples of thermal bridges include wood studs in wall assemblies, metal fasteners, and concrete slabs that extend from the interior to the exterior of the building. Thermal bridges can significantly reduce the overall thermal performance of the building envelope, even if the insulation itself has a high R-value.
To mitigate the effects of thermal bridging, several strategies can be employed, such as using thermally broken framing members, adding a continuous layer of insulation on the exterior of the building, and carefully detailing connections between building components. Advanced modeling tools, such as finite element analysis, can be used to identify and quantify the effects of thermal bridges, allowing designers to optimize the building envelope for thermal performance.
2.3 Heat Transfer Mechanisms
Heat transfer occurs through three primary mechanisms: conduction, convection, and radiation. Conduction is the transfer of heat through a solid material due to temperature differences. Convection is the transfer of heat through a fluid (liquid or gas) due to the movement of the fluid. Radiation is the transfer of heat through electromagnetic waves.
Insulation materials reduce heat transfer by minimizing conduction, convection, and radiation. For example, fibrous insulation materials, such as fiberglass and mineral wool, reduce conduction by creating a matrix of air pockets that inhibit heat flow. They also reduce convection by limiting the movement of air within the insulation. Reflective insulation materials, such as radiant barriers, reduce radiation by reflecting heat away from the building.
Many thanks to our sponsor Focus 360 Energy who helped us prepare this research report.
3. Types of Insulation Materials
3.1 Conventional Insulation Materials
- Fiberglass: Fiberglass is a widely used insulation material made from recycled glass. It is available in batts, rolls, and loose-fill forms. Fiberglass is relatively inexpensive and has good thermal performance, but it can be irritating to the skin and lungs during installation.
- Mineral Wool: Mineral wool is made from rock or slag and is also available in batts, rolls, and loose-fill forms. Mineral wool has similar thermal performance to fiberglass but is more fire-resistant and less irritating to handle.
- Cellulose: Cellulose is made from recycled paper and is typically used as loose-fill insulation. Cellulose is environmentally friendly and has good thermal performance, but it can be susceptible to moisture damage if not properly installed.
- Spray Foam: Spray foam insulation is made from polyurethane or polyisocyanurate and is applied as a liquid that expands to fill cavities. Spray foam has excellent thermal performance and can also act as an air barrier, but it is more expensive than other types of insulation.
3.2 Advanced Insulation Materials
- Vacuum Insulation Panels (VIPs): VIPs consist of a rigid core material encapsulated in a gas-tight envelope. The air is evacuated from the envelope, creating a vacuum that significantly reduces heat transfer. VIPs have extremely high R-values per inch of thickness but are also very expensive and fragile.
- Aerogels: Aerogels are highly porous materials with extremely low densities. They have excellent thermal performance and are also lightweight and fire-resistant. However, aerogels are currently expensive and are primarily used in niche applications.
- Phase Change Materials (PCMs): PCMs are materials that absorb and release heat as they undergo a phase change, such as melting or freezing. PCMs can be integrated into building materials to improve their thermal mass and reduce temperature fluctuations. PCMs are still under development, and their long-term performance and cost-effectiveness are still being evaluated.
- Reflective Insulation (Radiant Barriers): Radiant barriers are highly reflective materials that reduce heat transfer by reflecting radiant energy. They are typically used in attics and roofs to reduce cooling loads in hot climates. Radiant barriers are most effective when there is an air space between the reflective surface and the other building materials.
3.3 Considerations for Material Selection
The selection of insulation materials depends on a variety of factors, including:
- Thermal performance: The R-value or U-factor of the material.
- Cost: The initial cost of the material and the installation cost.
- Durability: The resistance of the material to moisture, pests, and degradation.
- Environmental impact: The embodied energy of the material, its recyclability, and its impact on indoor air quality.
- Installation requirements: The ease of installation and the required tools and equipment.
- Fire resistance: The fire rating of the material and its contribution to fire spread.
- Acoustic performance: The ability of the material to reduce noise transmission.
In my opinion, considering the holistic view, natural fiber based insulation such as sheep wool and hemp offer attractive options that are sustainable from manufacturing to end of life, while also delivering strong thermal performance and acoustic performance. A life-cycle cost analysis is advised to determine the optimal option for any particular building project.
Many thanks to our sponsor Focus 360 Energy who helped us prepare this research report.
4. Installation Techniques and Best Practices
4.1 Proper Installation is Critical
The effectiveness of insulation is highly dependent on proper installation. Gaps, voids, and compressions in the insulation can significantly reduce its thermal performance. It is essential to follow the manufacturer’s instructions and to use qualified installers to ensure that the insulation is properly installed.
4.2 Specific Insulation Types Installation Methods
- Batts and Rolls: Batts and rolls should be cut to fit snugly between framing members and should be installed without compressing the insulation. Gaps around electrical boxes and plumbing should be filled with insulation.
- Loose-Fill: Loose-fill insulation should be installed to the recommended density to achieve the specified R-value. Attic insulation should be evenly distributed and should not block ventilation openings.
- Spray Foam: Spray foam insulation should be applied by qualified installers who are trained in the proper application techniques. Spray foam should be applied in multiple layers to achieve the desired thickness and to avoid overheating.
- VIPs: VIPs should be installed carefully to avoid damage to the vacuum envelope. VIPs should be protected from moisture and should not be exposed to direct sunlight.
- Reflective Insulation: Radiant barriers should be installed with an air space between the reflective surface and other building materials. Radiant barriers should be installed with the reflective surface facing the direction of the heat source.
4.3 Air Sealing and Vapor Control
Air sealing and vapor control are essential components of a well-insulated building envelope. Air sealing reduces air leakage, which can significantly increase energy consumption and create drafts. Vapor control prevents moisture from entering the building envelope, which can lead to mold growth and structural damage.
Air sealing can be achieved by sealing cracks and gaps around windows, doors, and other penetrations in the building envelope. Vapor control can be achieved by installing a vapor retarder on the warm side of the insulation. In cold climates, the vapor retarder should be installed on the interior side of the insulation. In hot climates, the vapor retarder should be installed on the exterior side of the insulation.
It’s important to perform a hygrothermal analysis of a building envelope before installation, to ensure that condensation risk is managed to an acceptable level. Software such as WUFI provide these capabilities.
Many thanks to our sponsor Focus 360 Energy who helped us prepare this research report.
5. Cost Analysis and Life Cycle Assessment
5.1 Initial Cost vs. Long-Term Savings
The cost of insulation is an important consideration, but it is essential to consider the long-term savings that can be achieved through reduced energy consumption. A life cycle cost analysis (LCCA) can be used to compare the costs and benefits of different insulation options over the lifetime of the building.
5.2 Factors Affecting Cost
The cost of insulation depends on a variety of factors, including:
- Material type: Some insulation materials are more expensive than others.
- R-value: Higher R-value insulation typically costs more.
- Installation complexity: More complex installations typically cost more.
- Labor costs: Labor costs vary depending on the location and the skill of the installer.
- Quantity: Bulk purchases typically result in lower unit costs.
5.3 Environmental Impact Considerations
The environmental impact of insulation should also be considered. Some insulation materials have a higher embodied energy than others. Embodied energy is the total energy required to extract, process, manufacture, and transport a material. It’s also important to consider the recyclability of insulation materials and their impact on indoor air quality.
Life cycle assessment (LCA) is a comprehensive method for evaluating the environmental impacts of a product or process over its entire life cycle, from raw material extraction to disposal or recycling. LCA can be used to compare the environmental performance of different insulation options and to identify opportunities for reducing their environmental impact.
Many thanks to our sponsor Focus 360 Energy who helped us prepare this research report.
6. Environmental Impact and Sustainability
6.1 Embodied Energy
As mentioned earlier, embodied energy is a critical metric in assessing the environmental impact of insulation materials. Materials with high embodied energy require significant energy input during their production, contributing to greenhouse gas emissions and resource depletion. Selecting insulation materials with lower embodied energy, such as recycled or bio-based options, can significantly reduce the environmental footprint of a building.
6.2 Recyclability and Reusability
The recyclability and reusability of insulation materials are also important considerations. Some materials, such as fiberglass and mineral wool, can be recycled, reducing the need for virgin materials. Other materials, such as spray foam, are difficult to recycle and may end up in landfills. The development of closed-loop recycling systems for insulation materials is an important area of research.
6.3 Indoor Air Quality
Insulation materials can also impact indoor air quality. Some materials, such as fiberglass, can release volatile organic compounds (VOCs) that can cause respiratory problems. It is important to select insulation materials that are low-VOC or VOC-free. Proper ventilation is also essential to maintain good indoor air quality.
6.4 Climate Change Mitigation and Adaptation
Effective insulation plays a crucial role in mitigating climate change by reducing energy consumption and greenhouse gas emissions. It also contributes to climate change adaptation by making buildings more resilient to extreme weather events, such as heat waves and cold snaps. Buildings with high levels of insulation are better able to maintain comfortable indoor temperatures during power outages, reducing the risk of heat stress or hypothermia.
Many thanks to our sponsor Focus 360 Energy who helped us prepare this research report.
7. Future Trends and Research Directions
7.1 Nanomaterials
Nanomaterials, such as aerogels and nanofiber-based insulation, offer the potential for significant improvements in thermal performance. These materials have extremely high R-values per inch of thickness and can be used in applications where space is limited. Further research is needed to reduce the cost of nanomaterials and to improve their durability.
7.2 Bio-Based Materials
Bio-based insulation materials, such as hemp, sheep’s wool, and cellulose, are gaining popularity due to their low embodied energy and renewability. These materials offer a sustainable alternative to conventional insulation materials. Further research is needed to improve the performance and durability of bio-based insulation materials and to develop new applications for them.
7.3 Smart Insulation
Smart insulation materials are capable of changing their thermal properties in response to changes in temperature or humidity. For example, phase change materials (PCMs) can absorb and release heat as they undergo a phase change, helping to regulate indoor temperatures. Further research is needed to develop more effective and affordable smart insulation materials.
7.4 Integrated Design Approaches
Integrated design approaches, which consider the interactions between different building systems, are essential for achieving high-performance, sustainable building envelopes. This includes optimizing the orientation of the building, selecting appropriate window glazing, and integrating insulation with air sealing and ventilation systems. Advanced building modeling tools can be used to simulate the performance of different design options and to identify the most effective solutions.
7.5 Advanced Modeling and Simulation
Sophisticated modeling and simulation tools are increasingly important for predicting the performance of insulation materials and building envelopes under various conditions. These tools can account for complex heat transfer mechanisms, moisture transport, and the effects of thermal bridging. Further development of these tools is needed to improve their accuracy and usability.
Many thanks to our sponsor Focus 360 Energy who helped us prepare this research report.
8. Conclusion
Insulation is a critical component of sustainable building design, playing a vital role in reducing energy consumption, lowering greenhouse gas emissions, and improving indoor environmental quality. The selection and implementation of insulation strategies require careful consideration of various factors, including material properties, installation techniques, cost-effectiveness, and environmental impact. Advanced insulation materials and technologies, such as vacuum insulation panels, aerogels, and phase change materials, offer the potential for significant improvements in thermal performance, but their cost and durability remain challenges.
Future research should focus on developing innovative insulation materials, improving modeling tools, and promoting integrated design approaches to achieve high-performance, sustainable building envelopes. By embracing these advancements, the building sector can make a significant contribution to mitigating climate change and creating a more sustainable future.
Many thanks to our sponsor Focus 360 Energy who helped us prepare this research report.
References
- ASHRAE Handbook – Fundamentals
- U.S. Department of Energy – Building Energy Codes Program
- National Insulation Association
- Passive House Institute
- Vacuum Insulation Panel Association (VIPA)
- Jelle, B. P., & Hynd, A. W. (2011). Vacuum insulation panels (VIPs) for building applications: a review and future directions. Energy and Buildings, 43(10), 2577-2603.
- Baetens, R., Jelle, B. P., & Gustavsen, A. (2010). Aerogel insulation for building applications: A state-of-the-art review. Energy and Buildings, 43(4), 761-769.
- Cabeza, L. F., Castell, A., Barreneche, C., De Gracia, A., & Fernández, A. I. (2011). Materials used as PCM in thermal energy storage in buildings: A review. Renewable and Sustainable Energy Reviews, 15(3), 1675-1695.
- Hegger, M., Auch-Schwelk, A., Fuchs, M., & Rosenkranz, T. (2019). Baustoff-Atlas. Springer Vieweg.
- Dylewski, R., Adamczyk, J., & Szubel, M. (2023). Experimental assessment of the thermal performance of vacuum insulation panels in building envelope. Energy and Buildings, 281, 112721.
So, VIPs are like the ninjas of insulation – super effective but handle with extreme care. Wonder if we’ll see self-healing insulation someday, patching itself up after a rogue nail attack? That would be a game changer!