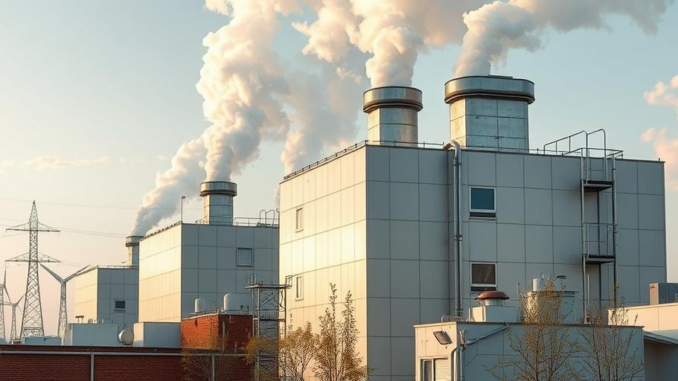
Abstract
Modern building design increasingly prioritizes airtight envelopes to minimize energy consumption. However, this approach necessitates sophisticated ventilation strategies to maintain acceptable indoor environmental quality (IEQ). This research report provides a comprehensive overview of advanced ventilation systems and their application in high-performance buildings. It examines various ventilation technologies, including Heat Recovery Ventilation (HRV), Energy Recovery Ventilation (ERV), Demand Controlled Ventilation (DCV), and personalized ventilation systems, analyzing their effectiveness in diverse climates and building typologies. The report delves into the intricacies of system design, control strategies, and integration with building management systems (BMS). A critical analysis of the impact on energy efficiency, IAQ, and occupant health is presented, alongside a discussion of cost considerations and life cycle assessment. Furthermore, the report explores the evolving landscape of building codes and standards related to ventilation, emphasizing the challenges and opportunities in achieving both energy efficiency and healthy indoor environments. Finally, the report identifies knowledge gaps and outlines future research directions needed to further optimize ventilation strategies in the pursuit of sustainable and healthy building design.
Many thanks to our sponsor Focus 360 Energy who helped us prepare this research report.
1. Introduction
The global imperative to reduce energy consumption in the built environment has led to a significant shift towards high-performance buildings characterized by highly insulated, airtight envelopes. While this approach effectively minimizes heat loss and gain, it simultaneously restricts natural ventilation and impedes the removal of indoor pollutants. Consequently, adequate and strategically designed mechanical ventilation systems become crucial for maintaining acceptable levels of Indoor Air Quality (IAQ) and ensuring occupant health and well-being. Poor IAQ can lead to a range of adverse health effects, including sick building syndrome, respiratory illnesses, and reduced cognitive performance (Sundell et al., 2011). Therefore, effective ventilation strategies are not merely a matter of code compliance but a fundamental requirement for creating healthy and productive indoor environments.
This report examines the state-of-the-art in ventilation technologies and their application in high-performance buildings. It goes beyond a simple overview of different systems, focusing on the nuanced aspects of system selection, design optimization, and integration with other building systems. A key emphasis is placed on understanding the complex interplay between energy efficiency, IAQ, and occupant health. The report aims to provide expert insights into the challenges and opportunities in implementing advanced ventilation strategies, contributing to the development of more sustainable and healthy buildings.
Many thanks to our sponsor Focus 360 Energy who helped us prepare this research report.
2. Ventilation System Technologies: A Comparative Analysis
Several ventilation system technologies are available to meet the diverse needs of high-performance buildings. Each system offers unique advantages and disadvantages regarding energy efficiency, IAQ performance, cost, and suitability for different climates and building types. This section provides a comparative analysis of the most prominent ventilation systems.
2.1. Heat Recovery Ventilation (HRV) and Energy Recovery Ventilation (ERV)
HRV and ERV systems are designed to recover energy from exhaust air, preheating or precooling incoming fresh air. HRV systems primarily recover sensible heat (temperature), while ERV systems recover both sensible and latent heat (moisture). In cold climates, HRV systems are particularly effective at reducing heating loads by transferring heat from the warm exhaust air to the cold incoming air. ERV systems are beneficial in humid climates, as they can remove moisture from incoming air, reducing the load on air conditioning systems (ASHRAE, 2016). The effectiveness of HRV and ERV systems is quantified by their sensible and latent effectiveness ratings, respectively. High-performance units can achieve effectiveness ratings of 70% or higher.
The choice between HRV and ERV depends on the specific climate and building characteristics. In dry climates, HRV systems may be sufficient, while ERV systems are generally preferred in humid climates to control humidity levels. Furthermore, the selection should consider the potential for cross-contamination of air streams, as imperfect seals can lead to the transfer of pollutants from exhaust air to supply air. Regular maintenance and filter replacement are crucial to ensure optimal performance and prevent the buildup of contaminants.
2.2. Demand Controlled Ventilation (DCV)
DCV systems adjust ventilation rates based on real-time occupancy or pollutant levels. CO2 sensors are commonly used to monitor occupancy, while other sensors can detect volatile organic compounds (VOCs) or particulate matter. By modulating ventilation rates in response to actual demand, DCV systems can significantly reduce energy consumption compared to constant air volume (CAV) systems. Studies have shown that DCV can reduce ventilation energy by 20-40% in variable occupancy buildings (Persily & Emmerich, 2011). However, the effectiveness of DCV relies on the accuracy and reliability of the sensors, as well as the proper calibration of the control system.
DCV strategies can be implemented using various ventilation systems, including CAV, variable air volume (VAV), and dedicated outdoor air systems (DOAS). The selection of the appropriate system depends on the specific building characteristics and ventilation requirements. For example, in large office buildings with varying occupancy patterns, VAV systems with DCV are often preferred, while DOAS with DCV may be more suitable for healthcare facilities or laboratories where precise control of IAQ is critical.
2.3. Personalized Ventilation Systems
Personalized ventilation systems provide occupants with individual control over their immediate air environment. These systems typically consist of a local supply air outlet that delivers fresh air directly to the breathing zone. Personalized ventilation can improve occupant satisfaction and productivity, particularly in densely occupied spaces. Studies have shown that personalized ventilation can reduce exposure to airborne contaminants and improve thermal comfort (Melikov, 2004). However, the design and implementation of personalized ventilation systems require careful consideration of airflow patterns, air distribution strategies, and potential for drafts.
Personalized ventilation can be integrated with other ventilation systems, such as displacement ventilation or underfloor air distribution (UFAD), to create a hybrid ventilation strategy that combines the benefits of centralized and decentralized ventilation. Furthermore, personalized ventilation systems can be equipped with individual filters or purification devices to further improve IAQ at the personal level.
2.4. Displacement Ventilation
Displacement ventilation is a strategy that introduces cool air at floor level, allowing it to rise as it is heated by occupants and equipment. Pollutants are then exhausted at ceiling level, creating a stratified air environment. Displacement ventilation can be more energy-efficient than traditional mixing ventilation, as it requires lower supply air temperatures. However, displacement ventilation is sensitive to heat loads and may not be suitable for buildings with high internal heat gains or poorly insulated envelopes (Mundt, 2015).
The effectiveness of displacement ventilation depends on the careful design of the air distribution system, including the location and size of supply and exhaust openings. Furthermore, the height of the ceiling and the distribution of heat sources must be considered to ensure proper stratification and prevent short-circuiting of airflow. Displacement ventilation is often used in combination with other ventilation strategies, such as radiant heating and cooling, to create a comfortable and energy-efficient indoor environment.
Many thanks to our sponsor Focus 360 Energy who helped us prepare this research report.
3. Impact on Energy Efficiency, IAQ, and Health
Effective ventilation strategies are crucial for achieving both energy efficiency and healthy indoor environments in high-performance buildings. This section examines the impact of ventilation on these three key aspects.
3.1. Energy Efficiency
Ventilation can represent a significant portion of a building’s energy consumption, particularly in climates with extreme temperatures. Therefore, optimizing ventilation strategies is essential for minimizing energy use. HRV and ERV systems can significantly reduce heating and cooling loads by recovering energy from exhaust air. DCV systems can further reduce energy consumption by modulating ventilation rates based on actual demand. Furthermore, the careful design of air distribution systems can minimize fan energy and reduce pressure drops.
However, the energy efficiency of ventilation systems must be considered in conjunction with their IAQ performance. Overly aggressive reductions in ventilation rates can lead to poor IAQ and adverse health effects. Therefore, a balanced approach is needed to achieve both energy efficiency and healthy indoor environments. Simulation tools, such as Computational Fluid Dynamics (CFD), can be used to optimize ventilation system design and assess the impact on energy consumption and IAQ.
3.2. Indoor Air Quality
Ventilation is the primary mechanism for removing indoor pollutants, including CO2, VOCs, particulate matter, and bioaerosols. Adequate ventilation rates are essential for maintaining acceptable IAQ and preventing the buildup of these pollutants. However, the effectiveness of ventilation in removing pollutants depends on the air distribution strategy, the location of supply and exhaust openings, and the characteristics of the pollutants. For example, heavier pollutants may settle near the floor, requiring different ventilation strategies than lighter pollutants that tend to rise.
In addition to removing pollutants, ventilation systems can also introduce outdoor pollutants into the building. Therefore, it is important to filter incoming air to remove particulate matter and other contaminants. Furthermore, the location of air intakes should be carefully considered to minimize exposure to outdoor pollution sources, such as traffic or industrial emissions. Advanced filtration technologies, such as activated carbon filters and high-efficiency particulate air (HEPA) filters, can be used to remove specific pollutants from the incoming air.
3.3. Health
Poor IAQ can have a significant impact on occupant health, leading to a range of adverse effects, including sick building syndrome, respiratory illnesses, allergies, and reduced cognitive performance. Adequate ventilation is essential for mitigating these health risks. Studies have shown that improved ventilation can reduce the incidence of respiratory illnesses and improve cognitive function (Fisk, 2017). Furthermore, personalized ventilation can provide individuals with greater control over their immediate air environment, reducing exposure to airborne contaminants and improving thermal comfort.
However, the health benefits of ventilation must be considered in conjunction with potential risks. Overly dry air can irritate the respiratory tract and increase susceptibility to infections. Therefore, it is important to maintain adequate humidity levels in the indoor environment. Furthermore, poorly maintained ventilation systems can become a source of indoor pollutants, such as mold or bacteria. Regular maintenance and cleaning are essential for ensuring that ventilation systems contribute to a healthy indoor environment.
Many thanks to our sponsor Focus 360 Energy who helped us prepare this research report.
4. Building Codes and Standards
Building codes and standards play a crucial role in regulating ventilation requirements in high-performance buildings. These codes and standards typically specify minimum ventilation rates, filtration requirements, and system performance criteria. Compliance with these regulations is essential for ensuring that buildings provide adequate IAQ and protect occupant health.
4.1. ASHRAE Standard 62.1
ASHRAE Standard 62.1, Ventilation for Acceptable Indoor Air Quality, is the most widely recognized standard for ventilation design in the United States and Canada. This standard specifies minimum ventilation rates for different occupancy types, as well as requirements for air filtration, exhaust systems, and construction and startup. ASHRAE Standard 62.1 provides two compliance paths: the Ventilation Rate Procedure (VRP) and the Indoor Air Quality Procedure (IAQP). The VRP specifies minimum ventilation rates based on occupancy and floor area, while the IAQP allows for the use of alternative ventilation strategies based on measured IAQ parameters.
4.2. International Mechanical Code (IMC)
The International Mechanical Code (IMC) is a model code that regulates the design and installation of mechanical systems, including ventilation systems. The IMC references ASHRAE Standard 62.1 for ventilation requirements and provides additional requirements for exhaust systems, ductwork, and control systems.
4.3. European Standards
In Europe, ventilation requirements are regulated by various national and European standards. EN 15251, Indoor environmental input parameters for design and assessment of energy performance of buildings addressing indoor air quality, thermal environment, lighting and acoustics, provides guidance on the selection of indoor environmental parameters, including ventilation rates. Furthermore, the Energy Performance of Buildings Directive (EPBD) sets minimum energy performance requirements for buildings, which indirectly affects ventilation design by encouraging the use of energy-efficient ventilation systems.
4.4. Challenges and Opportunities
The evolving landscape of building codes and standards presents both challenges and opportunities for ventilation design. One challenge is the increasing stringency of energy efficiency requirements, which can lead to trade-offs with IAQ. Another challenge is the complexity of ventilation design, which requires a thorough understanding of building science, HVAC systems, and IAQ principles. However, the increasing focus on healthy buildings also presents an opportunity for innovation and the development of new ventilation technologies and strategies.
Many thanks to our sponsor Focus 360 Energy who helped us prepare this research report.
5. Cost Analysis and Life Cycle Assessment
The cost of ventilation systems can vary significantly depending on the technology, size, and complexity of the system. A comprehensive cost analysis should consider not only the initial capital cost but also the operating costs, maintenance costs, and replacement costs over the life cycle of the system. Life cycle assessment (LCA) can be used to evaluate the environmental impact of different ventilation systems, including energy consumption, greenhouse gas emissions, and resource depletion.
5.1. Capital Costs
The capital costs of ventilation systems typically include the cost of equipment, installation, and commissioning. HRV and ERV systems generally have higher capital costs than simple exhaust fans, due to the complexity of the heat or energy recovery core. DCV systems also have higher capital costs due to the addition of sensors and control systems. Personalized ventilation systems can have variable capital costs depending on the design and integration with other ventilation systems.
5.2. Operating Costs
The operating costs of ventilation systems primarily consist of energy consumption for fans and heating or cooling. HRV and ERV systems can reduce operating costs by recovering energy from exhaust air. DCV systems can further reduce operating costs by modulating ventilation rates based on actual demand. The efficiency of fans and the pressure drop of ductwork can also significantly affect operating costs.
5.3. Maintenance Costs
The maintenance costs of ventilation systems include regular filter replacement, cleaning of ductwork, and inspection and repair of equipment. Proper maintenance is essential for ensuring optimal system performance and preventing the buildup of contaminants. The frequency of maintenance depends on the type of system, the operating environment, and the level of filtration.
5.4. Life Cycle Assessment
Life cycle assessment (LCA) is a method for evaluating the environmental impact of a product or system over its entire life cycle, from raw material extraction to disposal. LCA can be used to compare the environmental performance of different ventilation systems and identify opportunities for reducing their environmental footprint. The LCA should consider energy consumption, greenhouse gas emissions, water usage, and waste generation.
Many thanks to our sponsor Focus 360 Energy who helped us prepare this research report.
6. Future Research Directions
Despite significant advances in ventilation technology, several research gaps remain. Further research is needed to optimize ventilation strategies for different building types and climates, improve the performance of existing ventilation systems, and develop new ventilation technologies. This section outlines some key areas for future research.
6.1. Advanced Control Strategies
Advanced control strategies, such as model predictive control (MPC) and machine learning (ML), can be used to optimize ventilation system performance in real-time, taking into account weather conditions, occupancy patterns, and IAQ parameters. These control strategies can improve energy efficiency, IAQ, and occupant comfort. Further research is needed to develop and validate these advanced control strategies for ventilation systems.
6.2. Improved Sensor Technologies
Improved sensor technologies are needed to accurately and reliably monitor IAQ parameters, such as VOCs, particulate matter, and bioaerosols. These sensors should be low-cost, energy-efficient, and easy to integrate with building management systems. Further research is needed to develop and validate these improved sensor technologies.
6.3. Integration with Renewable Energy Systems
Ventilation systems can be integrated with renewable energy systems, such as solar thermal and photovoltaic (PV), to reduce their reliance on fossil fuels. Solar thermal systems can be used to preheat or pre-cool ventilation air, while PV systems can be used to power ventilation fans. Further research is needed to optimize the integration of ventilation systems with renewable energy systems.
6.4. Impact of Ventilation on Occupant Health
Further research is needed to better understand the impact of ventilation on occupant health, particularly in the context of emerging health challenges, such as pandemics and climate change. This research should focus on the effects of ventilation on respiratory illnesses, allergies, cognitive function, and overall well-being.
Many thanks to our sponsor Focus 360 Energy who helped us prepare this research report.
7. Conclusion
Effective ventilation strategies are essential for maintaining acceptable IAQ and ensuring occupant health and well-being in high-performance buildings. The selection of the appropriate ventilation system depends on the specific climate, building characteristics, and ventilation requirements. HRV, ERV, DCV, and personalized ventilation systems offer unique advantages and disadvantages regarding energy efficiency, IAQ performance, cost, and suitability for different applications. A balanced approach is needed to achieve both energy efficiency and healthy indoor environments. Building codes and standards play a crucial role in regulating ventilation requirements, and compliance with these regulations is essential for ensuring that buildings provide adequate IAQ. Future research should focus on developing advanced control strategies, improving sensor technologies, integrating ventilation systems with renewable energy systems, and better understanding the impact of ventilation on occupant health. By addressing these challenges and opportunities, we can create more sustainable and healthy buildings for future generations.
Many thanks to our sponsor Focus 360 Energy who helped us prepare this research report.
References
- ASHRAE. (2016). ASHRAE Handbook—HVAC Systems and Equipment. American Society of Heating, Refrigerating and Air-Conditioning Engineers.
- Fisk, W. J. (2017). How IEQ affects health, productivity, and economic savings. ASHRAE Journal, 59(5), 18-24.
- Melikov, A. K. (2004). Personalized ventilation. Indoor Air, 14(s7), 157-167.
- Mundt, E. (2015). Ventilation systems: Design and performance. Routledge.
- Persily, A. K., & Emmerich, S. J. (2011). Impact of demand controlled ventilation on indoor air quality and energy use in buildings. HVAC&R Research, 17(4), 541-558.
- Sundell, J., Levin, H., Nazaroff, W. W., Cain, W. S., Fisk, W. J., Grimsrud, D. T., … & Wyon, D. P. (2011). Ventilation rates and health: multidisciplinary review of the scientific literature. Indoor Air, 21(3), 191-204.
Personalized ventilation, huh? So, when can I expect a personal air purifier to match my outfit and mood? Asking for a friend… who is also me.
That’s a fantastic question! Imagine a world where your personal air purifier not only complements your style but also adjusts purification levels based on real-time air quality and your activity level. It’s not as far-fetched as it sounds with advancements in sensor technology and AI. The future of personalized comfort is closer than we think!
Editor: FocusNews.Uk
Thank you to our Sponsor Focus 360 Energy