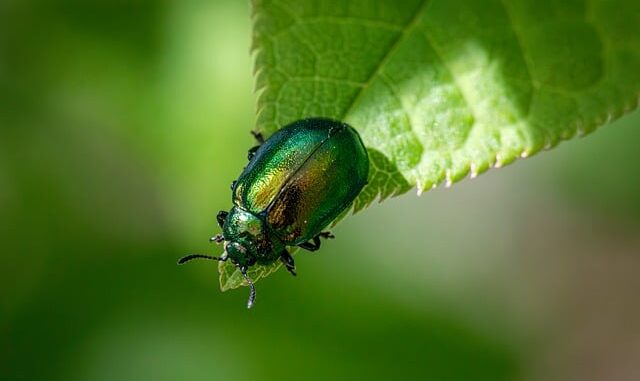
Abstract
This research report explores the evolving landscape of ecological science in the Anthropocene, a period marked by unprecedented human influence on the Earth’s ecosystems. It delves into the integration of ecological theory, cutting-edge technologies, and practical conservation strategies to address the escalating challenges of biodiversity loss and environmental degradation. We examine the limitations of traditional ecological frameworks in the face of rapid global change and advocate for a more holistic, interdisciplinary approach that incorporates socio-ecological perspectives. The report investigates the role of remote sensing, environmental DNA (eDNA) analysis, and artificial intelligence (AI) in advancing ecological monitoring and understanding. Furthermore, it critically assesses the effectiveness of current conservation practices and proposes novel strategies, including rewilding initiatives, ecosystem restoration projects, and the implementation of ecological engineering solutions. Finally, the report emphasizes the importance of adaptive management and stakeholder engagement in ensuring the long-term sustainability of conservation efforts. The overall aim is to provide a comprehensive overview of the current state of ecological science and to identify key areas for future research and innovation to safeguard biodiversity in a rapidly changing world.
Many thanks to our sponsor Focus 360 Energy who helped us prepare this research report.
1. Introduction: Ecology in the Anthropocene
Ecology, the study of the interactions between organisms and their environment, has always been a dynamic field. However, the current era, often termed the Anthropocene, presents unique and pressing challenges. The scale and pace of human impacts on the planet, including climate change, habitat destruction, pollution, and invasive species, are unprecedented, driving biodiversity loss at an alarming rate (Steffen et al., 2011). Traditional ecological frameworks, while foundational, are often insufficient to address the complexities of these challenges. A key limitation lies in their tendency to treat ecosystems as isolated entities, neglecting the pervasive influence of human activities and socio-ecological interactions.
This report argues for a paradigm shift in ecological science, one that embraces interdisciplinarity, technological innovation, and a more holistic understanding of the interconnectedness between human societies and natural systems. It critically examines the current state of ecological knowledge, exploring the strengths and weaknesses of existing theoretical frameworks and conservation practices. The report also investigates the potential of emerging technologies to enhance ecological monitoring and management. Furthermore, we emphasize the importance of adaptive management, which involves continually evaluating and adjusting conservation strategies based on new data and insights, and the critical role of stakeholder engagement in ensuring the long-term success of conservation efforts.
Many thanks to our sponsor Focus 360 Energy who helped us prepare this research report.
2. Ecological Theory: Evolving Frameworks for a Changing World
Classical ecological theory provides a crucial foundation for understanding ecosystem dynamics. Concepts such as carrying capacity, trophic interactions, and succession have shaped our understanding of how populations grow, communities are structured, and ecosystems function. However, these models often assume a degree of stability and predictability that is increasingly rare in the Anthropocene.
2.1 Limitations of Traditional Approaches
The assumption of equilibrium, for instance, is challenged by the prevalence of non-equilibrium dynamics driven by climate change and other anthropogenic stressors (Beisner et al., 2003). The focus on single-species or pairwise interactions often overlooks the complex web of indirect effects and cascading impacts that characterize real-world ecosystems. Moreover, the traditional emphasis on undisturbed ecosystems has limited our ability to understand and manage the ecological consequences of human activities in modified landscapes.
2.2 Emerging Theoretical Perspectives
To address these limitations, ecological science is increasingly incorporating new theoretical perspectives. These include:
- Socio-ecological systems theory: This framework recognizes the interconnectedness of social and ecological systems, emphasizing the feedback loops between human actions and environmental change (Berkes & Folke, 1998). It highlights the importance of considering social, economic, and political factors in ecological management.
- Resilience theory: This theory focuses on the capacity of ecosystems to absorb disturbance and reorganize while maintaining essential functions. It emphasizes the importance of promoting diversity, redundancy, and adaptive capacity to enhance resilience in the face of environmental change (Holling, 1973).
- Metacommunity ecology: This approach examines the dynamics of multiple local communities connected by dispersal. It recognizes that local community composition is influenced not only by local environmental conditions but also by regional processes such as species dispersal and colonization (Leibold et al., 2004).
These emerging perspectives provide a more nuanced and comprehensive understanding of ecosystem dynamics in the Anthropocene, guiding the development of more effective conservation strategies. For example, considering metacommunity dynamics can inform the design of protected area networks that facilitate species dispersal and maintain regional biodiversity. Adopting a resilience-based approach can help to identify vulnerable ecosystems and develop strategies to enhance their ability to withstand environmental shocks. Understanding socio-ecological systems allows for designing management plans that not only protect biodiversity but also improve the livelihoods of local communities.
Many thanks to our sponsor Focus 360 Energy who helped us prepare this research report.
3. Technological Advancements in Ecological Monitoring
Advancements in technology are revolutionizing ecological monitoring, providing unprecedented opportunities to collect data at finer spatial and temporal scales. These tools are essential for tracking environmental changes, assessing biodiversity, and evaluating the effectiveness of conservation efforts.
3.1 Remote Sensing
Remote sensing technologies, including satellite imagery, aerial photography, and LiDAR (Light Detection and Ranging), provide valuable information on habitat distribution, vegetation cover, and landscape structure (Turner, 1989). Satellite-based sensors can monitor changes in forest cover, track deforestation rates, and assess the impact of climate change on vegetation phenology. Aerial photography and LiDAR can provide detailed information on habitat structure, which is crucial for understanding species distributions and habitat suitability. The use of drones offers flexibility and high-resolution data acquisition, enabling researchers to monitor wildlife populations, assess habitat quality, and detect invasive species in remote areas.
3.2 Environmental DNA (eDNA) Analysis
eDNA analysis is a powerful tool for detecting the presence of species from trace amounts of DNA shed into the environment (Taberlet et al., 2012). This technique can be used to survey aquatic ecosystems, track invasive species, and monitor the biodiversity of soil communities. eDNA analysis is particularly useful for detecting rare or cryptic species that are difficult to observe using traditional methods. It offers a non-invasive and cost-effective approach to assess biodiversity and monitor the impact of environmental changes.
3.3 Acoustic Monitoring
Acoustic monitoring involves recording and analyzing environmental sounds to assess biodiversity and track animal behavior. This technique is particularly useful for monitoring birds, bats, and other vocalizing animals (Sueur et al., 2008). Automated acoustic monitoring systems can collect data continuously over long periods, providing insights into animal activity patterns and population trends. Acoustic monitoring can also be used to detect the presence of invasive species and assess the impact of noise pollution on wildlife.
3.4 Artificial Intelligence (AI) and Machine Learning
AI and machine learning algorithms are increasingly being used to analyze ecological data and make predictions about ecosystem dynamics. These techniques can be used to identify patterns in large datasets, classify habitats, and predict species distributions. AI-powered image recognition can automate the identification of species from camera trap images, reducing the time and effort required for manual analysis (Norouzzadeh et al., 2018). Machine learning algorithms can also be used to model the complex interactions between species and their environment, providing insights into the drivers of biodiversity change.
These technological advancements offer exciting opportunities to advance ecological research and improve conservation management. However, it is important to acknowledge the limitations of these technologies and to use them in conjunction with traditional ecological methods. The interpretation of remote sensing data requires ground-truthing to validate the accuracy of the results. eDNA analysis can be affected by factors such as DNA degradation and contamination, requiring careful experimental design and data analysis. AI algorithms can be biased if they are trained on incomplete or unrepresentative data. Therefore, it is crucial to combine technological tools with ecological expertise to ensure the reliability and validity of the results.
Many thanks to our sponsor Focus 360 Energy who helped us prepare this research report.
4. Conservation Strategies: Bridging the Gap Between Theory and Practice
Effective conservation requires the translation of ecological knowledge into practical management strategies. This involves understanding the threats to biodiversity, identifying priority areas for conservation, and implementing management actions that promote ecosystem health and resilience.
4.1 Protected Areas
Protected areas, such as national parks and reserves, are a cornerstone of biodiversity conservation. These areas provide refuge for endangered species, protect critical habitats, and maintain ecosystem services. However, the effectiveness of protected areas depends on their size, connectivity, and management (Watson et al., 2014). Small, isolated protected areas may not be sufficient to support viable populations of many species, especially in the face of climate change. Well-managed protected areas can provide significant benefits for biodiversity conservation, but they require adequate funding, staffing, and community support.
4.2 Ecosystem Restoration
Ecosystem restoration involves actively assisting the recovery of degraded ecosystems. This can include activities such as replanting forests, restoring wetlands, and removing invasive species. Ecosystem restoration is crucial for restoring ecosystem services, enhancing biodiversity, and mitigating the impacts of climate change (Gann et al., 2019). Successful restoration projects require a clear understanding of the ecological processes that drive ecosystem function and the identification of appropriate restoration techniques.
4.3 Rewilding
Rewilding is a conservation strategy that focuses on restoring natural processes and trophic interactions in degraded ecosystems. This often involves reintroducing keystone species, such as large herbivores and carnivores, to promote ecosystem health and resilience (Svenning et al., 2016). Rewilding can have significant benefits for biodiversity conservation, ecosystem services, and carbon sequestration. However, it can also be controversial, particularly when it involves reintroducing species that may conflict with human interests. Careful planning and stakeholder engagement are essential for successful rewilding projects.
4.4 Ecological Engineering
Ecological engineering is the design and construction of ecosystems to provide ecological services. This can include activities such as constructing artificial wetlands to treat wastewater, creating green roofs to reduce urban heat island effects, and building oyster reefs to protect coastlines from erosion. Ecological engineering can provide sustainable solutions to environmental problems while also enhancing biodiversity and ecosystem services (Mitsch & Jørgensen, 2003). However, it is important to carefully consider the potential impacts of ecological engineering projects on existing ecosystems and to ensure that they are designed to promote long-term ecological sustainability.
4.5 Adaptive Management and Stakeholder Engagement
Regardless of the specific conservation strategy employed, adaptive management and stakeholder engagement are crucial for long-term success. Adaptive management involves continually monitoring the outcomes of conservation actions and adjusting management strategies based on new data and insights. This allows for flexibility and learning in the face of uncertainty. Stakeholder engagement involves involving local communities, government agencies, and other relevant stakeholders in the planning and implementation of conservation projects. This can help to build support for conservation and ensure that management actions are aligned with local needs and priorities. Conservation efforts will be most effective when they are grounded in sound ecological science, supported by technological innovation, and implemented through adaptive management and stakeholder engagement.
Many thanks to our sponsor Focus 360 Energy who helped us prepare this research report.
5. Case Studies: Examples of Successful Ecological Interventions
Examining specific case studies can provide valuable insights into the practical application of ecological principles and the effectiveness of different conservation strategies.
5.1 Yellowstone National Park: Wolf Reintroduction
The reintroduction of wolves to Yellowstone National Park in 1995 is a classic example of a successful rewilding project. After being extirpated from the park in the early 20th century, wolves were reintroduced to restore top-down trophic control. The presence of wolves has had cascading effects on the ecosystem, reducing elk populations, promoting riparian vegetation growth, and increasing biodiversity (Ripple & Beschta, 2012). This case study demonstrates the importance of keystone species in maintaining ecosystem health and resilience.
5.2 Kissimmee River Restoration Project
The Kissimmee River Restoration Project in Florida is one of the largest river restoration projects in the world. The river was channelized in the 1960s for flood control, resulting in the loss of wetlands and degradation of aquatic habitat. The restoration project involves restoring the river’s original floodplain, reconnecting wetlands, and re-establishing natural flow patterns. This project has resulted in significant improvements in water quality, habitat availability, and fish and wildlife populations (Dahm et al., 1995). This case study demonstrates the potential of large-scale ecosystem restoration projects to reverse the impacts of human activities.
5.3 Costa Rica’s Payment for Ecosystem Services Program
Costa Rica’s Payment for Ecosystem Services (PES) program provides financial incentives to landowners who protect and restore forests. The program aims to promote forest conservation, carbon sequestration, and water quality improvement. Studies have shown that the PES program has been successful in reducing deforestation rates and enhancing ecosystem services (Pagiola, 2008). This case study demonstrates the effectiveness of market-based instruments for promoting environmental conservation.
These case studies highlight the diversity of approaches to ecological conservation and the importance of tailoring strategies to specific ecological and social contexts. They also underscore the need for long-term monitoring and evaluation to assess the effectiveness of conservation interventions and adapt management strategies as needed.
Many thanks to our sponsor Focus 360 Energy who helped us prepare this research report.
6. Future Directions: Challenges and Opportunities
Despite significant advances in ecological science and conservation practice, many challenges remain. Addressing these challenges will require continued innovation, collaboration, and a commitment to long-term sustainability.
6.1 Climate Change Impacts
Climate change is one of the most pressing threats to biodiversity, altering species distributions, disrupting ecological interactions, and increasing the frequency and intensity of extreme weather events. Future research needs to focus on understanding the impacts of climate change on ecosystems and developing strategies to mitigate these impacts. This includes identifying climate refugia, promoting climate-smart conservation, and enhancing ecosystem resilience to climate change.
6.2 Invasive Species
Invasive species continue to pose a major threat to biodiversity, outcompeting native species, altering ecosystem processes, and causing economic damage. Future research needs to focus on developing more effective methods for preventing the introduction and spread of invasive species, as well as controlling and eradicating established populations. This includes using eDNA analysis to detect invasive species early, developing targeted control methods, and restoring native ecosystems to enhance their resistance to invasion.
6.3 Urban Ecology
As urbanization continues to increase, it is important to understand the ecological impacts of cities and develop strategies to promote biodiversity in urban environments. Future research needs to focus on understanding the ecological processes that occur in cities, identifying opportunities to create and restore habitats, and promoting sustainable urban design and management. This includes creating green spaces, planting native vegetation, and reducing pollution.
6.4 Data Integration and Synthesis
The increasing availability of ecological data from diverse sources presents both opportunities and challenges. Future research needs to focus on developing methods for integrating and synthesizing data from different sources to gain a more comprehensive understanding of ecosystem dynamics. This includes developing data standards, creating data repositories, and using machine learning algorithms to analyze large datasets.
6.5 Interdisciplinary Collaboration
Addressing the complex challenges of biodiversity conservation requires interdisciplinary collaboration among ecologists, social scientists, economists, and policymakers. Future research needs to focus on fostering interdisciplinary collaborations and developing integrated solutions that address both ecological and social concerns. This includes promoting participatory research, engaging stakeholders in conservation planning, and developing policies that support sustainable development.
Many thanks to our sponsor Focus 360 Energy who helped us prepare this research report.
7. Conclusion
Ecology in the Anthropocene demands a proactive and adaptive approach. By integrating advanced technologies, refined theoretical frameworks, and practical conservation strategies, we can strive towards a future where biodiversity thrives alongside human development. The ongoing challenges of climate change, invasive species, and urbanization require innovative solutions, driven by data integration, interdisciplinary collaboration, and a steadfast commitment to adaptive management. Embracing these principles will be essential to ensuring the long-term health and resilience of our planet’s ecosystems.
Many thanks to our sponsor Focus 360 Energy who helped us prepare this research report.
References
- Beisner, B. E., Haydon, D. T., & Cuddington, K. (2003). Alternative stable states in ecology. Frontiers in Ecology and the Environment, 1(7), 376-382.
- Berkes, F., & Folke, C. (1998). Linking social and ecological systems: management practices and social mechanisms for building resilience. Cambridge University Press.
- Dahm, C. N., Cummins, K. W., Valett, H. M., & Coleman, R. L. (1995). An ecosystem view of the restoration of the Kissimmee River. Restoration Ecology, 3(3), 225-238.
- Gann, G. D., McDonald, T., Walder, B., Aronson, J., Nelson, C. R., Jonson, J., … & Hallett, J. G. (2019). International principles and standards for the practice of ecological restoration. Restoration Ecology, 27(S1), S1-S46.
- Holling, C. S. (1973). Resilience and stability of ecological systems. Annual Review of Ecology and Systematics, 4(1), 1-23.
- Leibold, M. A., Holyoak, M., Mouquet, N., Amarasekare, P., Chase, J. M., Hoopes, M. F., … & Loreau, M. (2004). The metacommunity concept: a framework for multi‐scale community ecology. Ecology Letters, 7(7), 601-613.
- Mitsch, W. J., & Jørgensen, S. E. (2003). Ecological engineering: a field of mutual benefit. Ecological Engineering, 20(5), 363-377.
- Norouzzadeh, M. S., Nguyen, A., Kosmala, M., Swanson, A., Palmer, M. S., Packer, C., & Clune, J. (2018). Automatically identifying, counting, and describing wild animals in camera-trap images with deep learning. Proceedings of the National Academy of Sciences, 115(25), E5716-E5725.
- Pagiola, S. (2008). Payments for environmental services in Costa Rica. Environmental Economics and Policy Studies, 9(2), 101-107.
- Ripple, W. J., & Beschta, R. L. (2012). Trophic cascades in Yellowstone: The first 15 years after wolf reintroduction. Biological Conservation, 145(1), 205-213.
- Steffen, W., Persson, Å., Deutsch, L., Zalasiewicz, J., Williams, M., Richardson, K., … & Crutzen, P. (2011). The Anthropocene: From global change to planetary stewardship. Ambio, 40(7), 739-761.
- Sueur, J., Aubin, T., & Simonis, C. (2008). Seewave: a free modular tool for sound analysis and synthesis. Bioacoustics, 18(2), 213-226.
- Svenning, J. C., Pedersen, P. B. M., Donlan, C. J., Ejrnæs, R., Faurby, S., Galetti, M., … & Vera, F. W. M. (2016). Science for rewilding: towards a paradigm shift in conservation. Frontiers in Ecology and the Environment, 14(2), 80-88.
- Taberlet, P., Coissac, E., Hajibabaei, M., & Rieseberg, L. H. (2012). Environmental DNA. Molecular Ecology, 21(8), 1789-1793.
- Turner, B. L. (1989). The human causes of global environmental change. The Earth as Transformed by Human Action: Global and Regional Changes in the Biosphere over the Past 300 Years, 11-40.
- Watson, J. E., Dudley, N., Segan, D. B., & Hockings, M. (2014). The performance and potential of protected areas. Nature, 515(7525), 67-73.
Rewilding, eh? If we reintroduce *all* the keystone species, does that mean I can finally get a pet saber-toothed tiger for my garden? Just kidding… mostly. Seriously though, what’s the plan for managing human-wildlife conflict in rewilding initiatives?
That’s a great question! Human-wildlife conflict is definitely a key consideration in rewilding. Mitigation strategies can range from habitat corridors to preventative measures like livestock protection and community education programs. Success relies on careful planning and collaboration with local communities. Let’s keep the conversation going!
Editor: FocusNews.Uk
Thank you to our Sponsor Focus 360 Energy
This report highlights the crucial role of interdisciplinary collaboration. Integrating socio-economic factors with ecological research can lead to more effective and sustainable conservation strategies, particularly when addressing complex issues like climate change impacts and urban ecology.
Thanks for highlighting the importance of interdisciplinary collaboration! It’s definitely key to tackling complex challenges. Considering socio-economic factors allows us to develop more sustainable and equitable solutions, especially when addressing issues like climate change and its effect on urban ecosystems. What innovative interdisciplinary approaches have you seen create positive results?
Editor: FocusNews.Uk
Thank you to our Sponsor Focus 360 Energy
The integration of AI and machine learning for ecological data analysis, particularly for identifying patterns in large datasets, holds immense potential for predictive modelling and conservation efforts. How can we ensure the accessibility and ethical application of these technologies in ecological research?
That’s a crucial point! Ensuring accessibility of AI in ecological research is vital. Perhaps open-source platforms and collaborative initiatives are key. Also, incorporating ethics training within ecological programs could ensure responsible application. What other measures could promote ethical use?
Editor: FocusNews.Uk
Thank you to our Sponsor Focus 360 Energy
So, if we’re rewilding, and AI’s helping us track everything, will it also learn to herd reintroduced megafauna away from my prize-winning petunias? Asking for a friend… who *might* have ordered a baby mammoth online.
That’s a fun thought! AI could potentially be trained to manage wildlife movement. Beyond petunias, imagine AI-driven systems optimizing habitat use to minimize human-wildlife conflict more broadly, using predictive modelling to suggest suitable corridors or buffer zones. We need to ensure that technology serves both ecological and community needs!
Editor: FocusNews.Uk
Thank you to our Sponsor Focus 360 Energy
The report’s emphasis on integrating socio-economic factors alongside ecological research is critical. How can we best measure and model the complex, bidirectional feedback loops between human well-being and ecosystem health to ensure conservation strategies are both effective and equitable?
That’s an excellent question! Quantifying those bidirectional relationships is a challenge, but vital. Perhaps developing integrated assessment models that incorporate both ecological and socio-economic indicators is the way forward. This would help decision-makers understand the potential trade-offs and synergies between conservation and human well-being. What specific indicators do you think would be most valuable to track?
Editor: FocusNews.Uk
Thank you to our Sponsor Focus 360 Energy
Given the increasing availability of ecological data, how might we best address potential biases or gaps in data collection to ensure that AI-driven analyses accurately reflect ecological realities and avoid skewed conservation priorities?
That’s a really insightful question! With so much data available, tackling biases is key. Perhaps a multi-pronged approach combining standardized protocols, diverse data sources, and AI algorithms designed for bias detection could help. It’s essential for responsible AI in conservation. What are your thoughts on incorporating citizen science data to broaden data collection?
Editor: FocusNews.Uk
Thank you to our Sponsor Focus 360 Energy
Considering the report’s mention of rewilding, could AI-driven predictive models be further developed to forecast the long-term ecological consequences of species reintroductions across diverse landscapes, especially concerning novel ecosystem dynamics?
That’s a fascinating question! AI’s potential for predictive modeling in rewilding is definitely huge. I agree novel ecosystem dynamics will need to be a core focus. Building on that, do you think incorporating real-time sensor data (e.g., weather patterns, animal movement) could further refine those AI models and create opportunities for adaptive management in the face of unpredictable change?
Editor: FocusNews.Uk
Thank you to our Sponsor Focus 360 Energy
The report rightly highlights the potential of eDNA analysis. Considering its sensitivity, how can we standardize eDNA collection and analysis protocols across different ecosystems to ensure data comparability and reliability for broad-scale biodiversity assessments?
That’s a great point! Standardization is definitely key for reliable eDNA analysis. Perhaps a centralized repository of validated primer sets and analysis pipelines could help? Also, incorporating internal controls within each sample could improve data quality. What are your thoughts on data sharing initiatives to improve standardization?
Editor: FocusNews.Uk
Thank you to our Sponsor Focus 360 Energy
That rewilding section had me hooked! Keystone species are cool and all, but what about reintroducing extinct ecosystem engineers like beavers to restore wetlands? Could AI help us predict the optimal placement and density for maximum impact? I’m picturing AI-powered beaver dams… for science!
That’s a fantastic point about beavers! AI-powered placement could revolutionize wetland restoration, optimizing dam locations for maximum ecological benefit. It’s exciting to think about the potential for technology to work alongside natural processes in such innovative ways. Perhaps future simulations could even account for climate change impacts on water flow. #Rewilding #AIConservation
Editor: FocusNews.Uk
Thank you to our Sponsor Focus 360 Energy