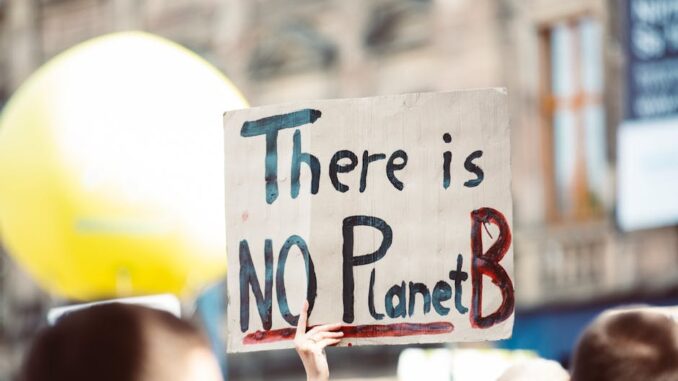
Abstract
Climate change presents a significant and multifaceted challenge to the built environment, particularly concerning indoor overheating. This report examines the complex interplay between projected climate scenarios, the resulting impact on indoor thermal comfort, the vulnerability of specific populations, and the imperative for implementing effective adaptation strategies. Focusing on the UK context, the report analyzes future climate projections pertaining to temperature increases and heatwave characteristics, assessing their implications for the thermal performance of buildings. Furthermore, it investigates the differential vulnerability of various demographic groups to overheating risks, emphasizing the socio-economic factors that exacerbate exposure and impact. The report proposes and evaluates a range of adaptation strategies, encompassing building design and retrofitting, urban planning interventions, behavioral adjustments, and policy frameworks. Ultimately, the report argues for a holistic and proactive approach to address overheating in the built environment, advocating for the integration of climate resilience into long-term planning and policy development to safeguard public health and well-being.
Many thanks to our sponsor Focus 360 Energy who helped us prepare this research report.
1. Introduction
Climate change is no longer a distant threat but a tangible reality manifesting across the globe. Rising global average temperatures, altered precipitation patterns, and the increased frequency and intensity of extreme weather events are already impacting human societies and natural ecosystems [1]. The built environment, which encompasses residential, commercial, and industrial structures, plays a dual role in this context. On the one hand, it is a significant contributor to greenhouse gas emissions through energy consumption and material production. On the other hand, it is highly susceptible to the adverse impacts of climate change, including flooding, storms, and, increasingly, overheating [2].
Overheating, defined as a sustained period of excessively high indoor temperatures, poses a serious threat to human health and well-being. Prolonged exposure to high temperatures can lead to heat stress, dehydration, heatstroke, and even death, particularly among vulnerable populations such as the elderly, children, and individuals with pre-existing health conditions [3]. The increasing prevalence of overheating in homes and other buildings is a direct consequence of rising ambient temperatures, coupled with factors such as inadequate building design, poor insulation, and lack of ventilation [4].
This report focuses on the specific challenge of overheating in the UK context, where climate change projections indicate a significant increase in average temperatures and a greater frequency of heatwaves. Understanding the potential impacts of these changes on indoor thermal comfort, identifying vulnerable populations, and developing effective adaptation strategies are crucial for mitigating the risks associated with overheating and ensuring the resilience of the built environment. The report adopts a multidisciplinary approach, drawing on climate science, building physics, public health, and urban planning to provide a comprehensive assessment of the issue and to inform policy recommendations.
Many thanks to our sponsor Focus 360 Energy who helped us prepare this research report.
2. Climate Change Projections and Their Implications for the UK
Detailed climate projections provide a crucial foundation for understanding the future risks associated with overheating. The UK Climate Projections (UKCP) provide the most comprehensive and up-to-date assessment of future climate scenarios for the UK, utilizing sophisticated climate models and incorporating various greenhouse gas emission pathways [5].
The key findings of UKCP18, the latest iteration of the projections, indicate a clear trend towards warmer temperatures across the UK. Under a high emissions scenario, average summer temperatures could increase by 3°C to 6°C by the end of the 21st century [6]. Furthermore, the frequency and intensity of heatwaves are projected to increase significantly. Heatwaves that were previously rare events are likely to become more common, with some projections suggesting that heatwaves similar to the 2003 European heatwave could occur every other year by the 2050s [7].
The implications of these changes for the built environment are profound. Rising ambient temperatures will directly increase the heat load on buildings, leading to higher indoor temperatures. The increased frequency and duration of heatwaves will exacerbate this effect, potentially resulting in prolonged periods of extreme indoor heat [8]. The thermal performance of buildings is directly affected by the external climate, with poorly insulated buildings and those lacking effective ventilation being particularly vulnerable to overheating. This is particularly true for older housing stock, which often lacks modern insulation and cooling systems.
The projected changes in precipitation patterns also have indirect implications for overheating. While the overall trend is towards drier summers in many parts of the UK, there is also an increased risk of heavy rainfall events. This combination of drier summers and more intense rainfall can lead to water stress and drought conditions, limiting the availability of water for evaporative cooling systems [9]. Furthermore, increased humidity, which can occur even in a generally drying climate, can exacerbate the effects of high temperatures, making it more difficult for the human body to regulate its temperature through sweating.
It is crucial to note that climate projections are inherently uncertain, and the actual changes that occur will depend on future greenhouse gas emissions and the complex interactions within the climate system. However, the overwhelming scientific consensus is that the UK will experience significant warming and an increase in heatwave frequency and intensity, making it imperative to prepare the built environment for these changes.
Many thanks to our sponsor Focus 360 Energy who helped us prepare this research report.
3. Impact on Indoor Environments and Thermal Comfort
The increasing frequency and intensity of heatwaves directly impact the indoor environment, affecting thermal comfort, health, and productivity. The degree of impact is contingent on various factors, including building design, materials, ventilation, and occupant behavior [10].
Traditional building designs in the UK, particularly older housing stock, were not designed to cope with the projected increases in temperature. Many homes lack adequate insulation, leading to rapid heat gain during hot weather and poor heat retention during cooler periods. Insufficient ventilation exacerbates the problem by trapping heat indoors, creating stagnant and uncomfortable conditions [11]. The use of materials with high thermal mass, such as concrete and brick, can also contribute to overheating, as these materials absorb and retain heat over extended periods [12].
The impact of overheating on thermal comfort can be quantified using various metrics, such as Predicted Mean Vote (PMV) and Predicted Percentage Dissatisfied (PPD). These metrics take into account factors such as air temperature, humidity, radiant temperature, air velocity, and clothing insulation to assess the overall level of thermal comfort. Studies have shown that even moderate increases in indoor temperature can lead to significant reductions in thermal comfort, particularly for individuals engaged in sedentary activities [13].
Beyond thermal comfort, overheating can have serious consequences for health. Prolonged exposure to high indoor temperatures can lead to heat stress, characterized by symptoms such as fatigue, dizziness, headache, and nausea. In severe cases, heat stress can progress to heatstroke, a life-threatening condition that requires immediate medical attention. The elderly, children, and individuals with chronic illnesses are particularly vulnerable to the health effects of overheating [14].
Overheating can also negatively impact cognitive performance and productivity. Studies have shown that high indoor temperatures can impair concentration, memory, and decision-making abilities [15]. This can have significant implications for workplaces, schools, and other environments where cognitive performance is critical. In residential settings, overheating can disrupt sleep patterns, leading to fatigue and reduced well-being [16].
The indoor environment is not only impacted by external climate but also by internal heat gains from occupants, equipment, and lighting. These internal heat sources can further exacerbate overheating, particularly in densely occupied buildings with high equipment density [17]. Reducing internal heat gains through energy-efficient appliances and lighting can help to mitigate the risk of overheating.
Many thanks to our sponsor Focus 360 Energy who helped us prepare this research report.
4. Vulnerability of Specific Populations
While overheating poses a threat to the general population, certain demographic groups are disproportionately vulnerable to its adverse effects. Understanding the factors that contribute to vulnerability is crucial for developing targeted interventions and ensuring that adaptation strategies are equitable and effective [18].
The elderly are particularly vulnerable to overheating due to age-related physiological changes that impair their ability to regulate body temperature. Older adults often have reduced sweating capacity, decreased thirst sensation, and increased susceptibility to dehydration. Furthermore, many elderly individuals have pre-existing health conditions, such as cardiovascular disease and respiratory illnesses, that can be exacerbated by heat stress [19]. Social isolation is also a significant risk factor for elderly individuals, as they may be less likely to seek help or access cooling resources during heatwaves [20].
Children are also highly vulnerable to overheating, as their bodies are less efficient at regulating temperature than adults. Infants and young children have a higher surface area-to-volume ratio, making them more susceptible to heat gain from the environment. They also rely on caregivers to provide adequate hydration and cooling [21].
Individuals with chronic illnesses, such as cardiovascular disease, respiratory illnesses, diabetes, and mental health conditions, are at increased risk of heat-related morbidity and mortality. These conditions can impair the body’s ability to cope with heat stress and can be exacerbated by high temperatures [22]. Certain medications can also increase susceptibility to heat-related illness.
Low-income households are often disproportionately affected by overheating due to a combination of factors, including poor housing quality, limited access to air conditioning, and higher energy costs. Low-income housing is often older and less well-insulated, making it more prone to overheating. Furthermore, low-income households may be less able to afford air conditioning or to pay for the increased energy costs associated with cooling [23]. Fuel poverty, where households struggle to afford adequate heating and cooling, can further exacerbate the vulnerability of low-income populations to overheating.
Geographic location can also influence vulnerability to overheating. Urban areas tend to experience higher temperatures than rural areas due to the urban heat island effect, where buildings and pavements absorb and retain heat. Residents of urban areas, particularly those living in densely populated areas with limited green space, are therefore at greater risk of overheating [24].
Addressing the vulnerability of specific populations requires a multifaceted approach that takes into account their unique needs and circumstances. Targeted interventions, such as providing cooling centers, distributing fans, and offering educational materials, can help to protect vulnerable individuals during heatwaves. Improving housing quality and reducing energy costs can also help to mitigate the long-term risks associated with overheating. Crucially, social support networks and community-based initiatives can play a vital role in identifying and assisting vulnerable individuals during periods of extreme heat.
Many thanks to our sponsor Focus 360 Energy who helped us prepare this research report.
5. Adaptation Strategies: Building Design and Retrofitting
Adaptation strategies are essential for mitigating the risks associated with overheating and ensuring the resilience of the built environment. These strategies can be broadly categorized into building design and retrofitting, urban planning interventions, behavioral adjustments, and policy frameworks [25].
Building design plays a crucial role in determining the thermal performance of buildings and their susceptibility to overheating. Incorporating passive design strategies, which minimize the need for mechanical cooling, is a key principle of climate-responsive building design [26]. These strategies include:
- Orientation: Orienting buildings to minimize solar gain during the hottest parts of the day can significantly reduce the heat load on the building. South-facing facades are particularly susceptible to solar gain during the summer months, while east- and west-facing facades can also experience significant heat gain [27].
- Shading: Providing shading to windows and walls can effectively block direct sunlight and reduce heat gain. Shading devices can include overhangs, awnings, shutters, and trees [28]. The effectiveness of shading devices depends on their design, orientation, and the time of year.
- Insulation: Proper insulation reduces the transfer of heat through the building envelope, keeping buildings cooler in summer and warmer in winter. High-performance insulation materials can significantly improve the thermal performance of buildings [29].
- Ventilation: Natural ventilation can effectively remove excess heat from buildings and improve indoor air quality. Cross-ventilation, where air flows through the building from one side to the other, is particularly effective at cooling buildings [30]. The design of windows and other openings should maximize natural ventilation opportunities.
- Materials: The choice of building materials can also influence thermal performance. Materials with high thermal mass, such as concrete and brick, can absorb and store heat, potentially contributing to overheating. However, they can also provide thermal inertia, moderating temperature fluctuations and reducing the need for mechanical cooling if well managed. Reflective materials, such as light-colored roofs and walls, can reduce solar absorption and keep buildings cooler [31].
Retrofitting existing buildings to improve their thermal performance is also essential for addressing overheating risks. Retrofitting measures can include:
- Insulation: Adding insulation to walls, roofs, and floors can significantly reduce heat transfer and improve the thermal performance of existing buildings [32].
- Window upgrades: Replacing single-pane windows with double- or triple-pane windows can reduce heat gain and improve insulation [33]. Low-emissivity (low-E) coatings on windows can further reduce heat gain.
- Shading: Installing shading devices, such as awnings or shutters, can reduce solar gain and improve thermal comfort [34].
- Ventilation improvements: Improving natural ventilation by adding or modifying windows and other openings can help to remove excess heat [35].
- Cool roofs: Applying a reflective coating to the roof can reduce solar absorption and keep the building cooler [36].
In addition to passive design strategies and retrofitting measures, mechanical cooling systems, such as air conditioning and evaporative coolers, can be used to provide supplemental cooling. However, these systems consume energy and can contribute to greenhouse gas emissions. It is therefore important to use energy-efficient cooling systems and to minimize their use through effective passive design and behavioral strategies [37].
Many thanks to our sponsor Focus 360 Energy who helped us prepare this research report.
6. Adaptation Strategies: Urban Planning and Policy Implications
Beyond individual building design and retrofitting, urban planning plays a critical role in mitigating overheating at the community and city-wide scales. Policy frameworks are essential for guiding and incentivizing adaptation measures across all sectors [38].
Urban planning strategies that can help to reduce overheating include:
- Green infrastructure: Increasing the amount of green space in urban areas, such as parks, gardens, and street trees, can help to cool the environment through evapotranspiration. Green spaces also provide shade and reduce the urban heat island effect [39].
- Urban density: Managing urban density to avoid overly dense development patterns can improve ventilation and reduce the concentration of heat. Encouraging mixed-use development can also reduce the need for long-distance travel and associated emissions [40].
- Building orientation and spacing: Planning new developments to optimize building orientation and spacing can maximize natural ventilation and minimize solar gain. Building codes can be used to regulate building orientation and spacing [41].
- Transportation planning: Promoting sustainable transportation modes, such as walking, cycling, and public transit, can reduce greenhouse gas emissions and improve air quality. Providing shaded walkways and bike paths can encourage active transportation even during hot weather [42].
- Water management: Implementing water management strategies, such as rainwater harvesting and permeable pavements, can reduce water stress and provide water for evaporative cooling [43].
Policy frameworks are essential for driving the adoption of adaptation strategies across all sectors. These frameworks can include:
- Building codes and regulations: Updating building codes to incorporate climate change considerations, such as requiring improved insulation, shading, and ventilation, can ensure that new buildings are resilient to overheating. Retrofitting requirements can also be introduced for existing buildings [44].
- Incentive programs: Providing financial incentives, such as tax credits and rebates, can encourage homeowners and businesses to invest in energy-efficient upgrades and adaptation measures [45].
- Public awareness campaigns: Educating the public about the risks of overheating and the steps they can take to protect themselves can help to reduce heat-related illness and mortality [46].
- Emergency preparedness plans: Developing and implementing emergency preparedness plans for heatwaves can help to ensure that vulnerable populations are protected and that resources are available to respond to heat emergencies [47].
- Climate risk assessments: Conducting regular climate risk assessments can help to identify vulnerabilities and prioritize adaptation measures [48].
- National adaptation strategies: Developing national adaptation strategies that set out clear goals and targets for climate resilience can provide a framework for coordinating adaptation efforts across all sectors [49].
The effectiveness of adaptation strategies depends on their implementation and enforcement. It is therefore crucial to ensure that policies are well-designed, adequately funded, and effectively enforced. Collaboration between government agencies, businesses, community organizations, and individuals is essential for achieving climate resilience.
Many thanks to our sponsor Focus 360 Energy who helped us prepare this research report.
7. Long-Term Planning and Policy Implications
Addressing overheating risks in the built environment requires a long-term perspective and a proactive approach. Climate change is an ongoing process, and the impacts of overheating will likely become more severe in the future. Therefore, it is essential to integrate climate resilience into long-term planning and policy development across all sectors [50].
Long-term planning should consider the following:
- Future climate scenarios: Planners should use the latest climate projections to assess the potential impacts of climate change on overheating risks and to develop adaptation strategies that are robust under a range of future scenarios [51].
- Infrastructure investments: Infrastructure investments, such as energy grids, water systems, and transportation networks, should be designed to be resilient to climate change impacts, including extreme heat [52].
- Land-use planning: Land-use planning should prioritize the development of green spaces, the preservation of natural areas, and the reduction of urban sprawl. This can help to reduce the urban heat island effect and improve air quality [53].
- Building codes and regulations: Building codes and regulations should be regularly updated to reflect the latest knowledge about climate change and best practices for climate-responsive building design [54].
- Community engagement: Engaging with communities in the planning process can help to ensure that adaptation strategies are tailored to local needs and priorities [55].
Policy implications include:
- Carbon reduction targets: Setting ambitious carbon reduction targets can help to mitigate the long-term drivers of climate change and reduce the severity of future warming [56].
- Energy efficiency standards: Implementing energy efficiency standards for buildings, appliances, and equipment can help to reduce energy consumption and greenhouse gas emissions [57].
- Renewable energy incentives: Providing incentives for the development and deployment of renewable energy technologies can help to reduce reliance on fossil fuels and transition to a cleaner energy system [58].
- Climate adaptation funding: Allocating sufficient funding for climate adaptation initiatives can help to ensure that adaptation strategies are effectively implemented and that vulnerable populations are protected [59].
- International cooperation: Collaborating with other countries to share knowledge, resources, and best practices can help to accelerate the global transition to a climate-resilient future [60].
The challenge of overheating in the built environment is complex and multifaceted. However, by taking a proactive and integrated approach, it is possible to mitigate the risks associated with overheating and to create a more sustainable and resilient future. This requires a commitment to long-term planning, policy development, and community engagement, as well as a willingness to embrace innovative technologies and design strategies. The goal should be to ensure that all individuals have access to safe, healthy, and comfortable indoor environments, regardless of their age, income, or geographic location.
Many thanks to our sponsor Focus 360 Energy who helped us prepare this research report.
8. Conclusion
Climate change poses a significant threat to the built environment, with overheating emerging as a critical concern. This report has highlighted the projected increases in temperature and heatwave frequency, emphasizing the impact on indoor environments and the vulnerability of specific populations. The adaptation strategies discussed, encompassing building design, urban planning, and policy interventions, offer a pathway towards mitigating these risks. However, the successful implementation of these strategies hinges on a holistic and proactive approach, integrating climate resilience into long-term planning and policy development.
While significant progress has been made in understanding the science of climate change and its potential impacts, further research is needed to refine climate projections, assess the effectiveness of adaptation strategies, and develop innovative solutions for mitigating overheating risks. Collaboration between researchers, policymakers, and practitioners is essential for translating research findings into practical applications. Ultimately, addressing the challenge of overheating requires a collective effort to create a more sustainable, resilient, and equitable built environment that protects public health and well-being in a changing climate.
Many thanks to our sponsor Focus 360 Energy who helped us prepare this research report.
References
[1] IPCC, 2021: Climate Change 2021: The Physical Science Basis. Contribution of Working Group I to the Sixth Assessment Report of the Intergovernmental Panel on Climate Change [Masson-Delmotte, V., P. Zhai, A. Pirani, S. L. Connors, C. Péan, S. Berger, N. Caud, Y. Chen, L. Goldfarb, M. I. Gomis, M. Huang, K. Leitzell, E. Lonnoy, J.B.R. Matthews, T. K. Maycock, T. Waterfield, O. Yelekçi, R. Yu, and B. Zhou (eds.)]. Cambridge University Press, Cambridge, United Kingdom and New York, NY, USA, 2391 pp. doi:10.1017/9781009157896
[2] Santamouris, M. (2014). Cooling the buildings – A review of passive and low-energy techniques. Energy and Buildings, 70, 477-493.
[3] Kovats, R. S., & Hajat, S. (2008). Heat stress and public health: a review. Annual Review of Public Health, 29, 41-55.
[4] Hajat, S., & Kosatky, T. (2010). Heat-related mortality: a review and exploration of heterogeneity. Journal of Epidemiology and Community Health, 64(9), 753-760.
[5] UK Climate Projections (UKCP18). (n.d.). Retrieved from https://www.metoffice.gov.uk/research/approach/collaboration/ukcp/index
[6] Murphy, J.M., et al. (2018). UKCP18 Science Overview Report. Met Office Hadley Centre.
[7] Kendon, E. J., et al. (2018). UKCP Local (2.2km) probabilistic climate change projections. Met Office Hadley Centre.
[8] Firth, S. K., Lomas, K. J., & Giridharan, R. (2013). A methodology for assessing the potential impacts of climate change on overheating in UK dwellings. Building Services Engineering Research and Technology, 34(1), 80-95.
[9] Watts, G., Allen, R., & Hannaford, J. (2015). UK Hydrological Outlook. Hydrology Research, 46(3), 447-450.
[10] Nicol, F., & Humphreys, M. (2002). Adaptive thermal comfort and sustainable thermal standards for buildings. Energy and Buildings, 34(6), 563-572.
[11] Gupta, R., Gregg, M., & Irving, A. (2012). Mainstreaming climate change adaptation in the UK housing sector: barriers, drivers, and policy implications. Building Research & Information, 40(5), 529-546.
[12] Givoni, B. (1998). Climate considerations in building and urban design. John Wiley & Sons.
[13] Fanger, P. O. (1970). Thermal comfort: Analysis and applications in environmental engineering. McGraw-Hill.
[14] Anderson, G. B., & Bell, M. L. (2009). Weather-related mortality: how heat, cold, and heat waves affect mortality in the United States. Epidemiology, 20(2), 205-213.
[15] Lan, L., Wargocki, P., Lian, Z., & Cheng, Y. (2011). Effects of moderate thermal stress on students’ performance in classrooms. Indoor Air, 21(5), 369-380.
[16] Lan, L., & Lian, Z. (2009). Use of indices in the evaluation of indoor environment. Energy and Buildings, 41(10), 1087-1091.
[17] Perez-Lombard, L., Ortiz, J., Pout, C., & Coronel, J. F. (2008). A review on buildings energy consumption information. Energy and Buildings, 40(3), 394-398.
[18] Harlan, S. L., Brazel, A. J., Prashad, S., Stefanov, W. L., & Larsen, L. (2006). Neighborhood microclimates and vulnerability to heat stress. Social Science & Medicine, 63(10), 2483-2499.
[19] Kenney, W. L., Hodgson, J. L., & LaBelle, R. G. (2014). Physiology of aging: A systems perspective. Comprehensive Physiology, 4(2), 761-832.
[20] Klinenberg, E. (2002). Heat wave: A social autopsy of disaster in Chicago. University of Chicago Press.
[21] Basu, R., & Samet, J. M. (2002). Relation between elevated ambient temperature and mortality in nine California counties. Epidemiology, 13(3), 265-272.
[22] Bouchama, A., & Knochel, J. P. (2002). Heat stroke. New England Journal of Medicine, 346(25), 1978-1988.
[23] Wolf, J., & McGregor, D. (2013). Climate change and social vulnerability: The case of fuel poverty in the UK. Global Environmental Change, 23(6), 1677-1685.
[24] Oke, T. R. (1982). The energetic basis of the urban heat island. Quarterly Journal of the Royal Meteorological Society, 108(455), 1-24.
[25] IPCC, 2014: Climate Change 2014: Impacts, Adaptation, and Vulnerability. Part A: Global and Sectoral Aspects. Contribution of Working Group II to the Fifth Assessment Report of the Intergovernmental Panel on Climate Change [Field, C.B., V.R. Barros, D.J. Dokken, K.J. Mach, M.D. Mastrandrea, T.E. Bilir, M. Chatterjee, K.L. Ebi, Y.O. Estrada, R.C. Genova, B. Girma, E.S. Kissel, A.N. Levy, S. MacCracken, P.R. Mastrandrea, and L.L. White (eds.)]. Cambridge University Press, Cambridge, United Kingdom and New York, NY, USA, 1132 pp.
[26] Watson, D., & Labs, K. (1993). Climatic design: Energy-efficient building principles and practices. McGraw-Hill.
[27] Olgyay, V. (1963). Design with climate: Bioclimatic approach to architectural regionalism. Princeton University Press.
[28] Brown, G. Z., & DeKay, M. (2001). Sun, wind, and light: Architectural design strategies. John Wiley & Sons.
[29] Kosny, J., & Christian, J. (2016). Retrofitting for energy efficiency. McGraw-Hill Education.
[30] Etheridge, D. W. (2011). Natural ventilation of buildings: theory, measurement and design. John Wiley & Sons.
[31] Doulos, L., Kolokotsa, D., & Catsaros, N. (2004). Influence of roof surface reflectance on the thermal behaviour of buildings. Solar Energy, 76(6), 681-695.
[32] Oreszczyn, T., & Lowe, R. (2010). How to achieve a low-carbon building stock: A UK perspective. Building Research & Information, 38(5), 503-517.
[33] Palmer, J., Cooper, I., & Boardman, B. (2005). Investigating the impact of double glazing and wall insulation on the indoor environment and energy consumption of English homes. Energy and Buildings, 37(10), 1093-1102.
[34] Li, D. H. W., & Lam, J. C. (2000). Investigation of the effects of shading devices on air-conditioning energy consumption in a high-rise office building in Hong Kong. Energy, 25(6), 579-594.
[35] Cook, D. (2002). Improving indoor air quality. Thomas Telford.
[36] Akbari, H., Rose, L. S., & Taha, H. (1999). Characterizing the fabric of the Sacramento urban heat island. Lawrence Berkeley National Laboratory, LBNL-42927.
[37] Perez-Lombard, L., Ortiz, J., & Pout, C. (2008). A review on calculation methods of energy performance indicators in buildings. Energy and Buildings, 40(3), 327-338.
[38] Beatley, T. (2016). Handbook of sustainable urbanism. Routledge.
[39] Bowler, D. E., Buyung-Ali, L. M., Knight, T. M., Pullin, A. S., & Sanderson, I. R. (2010). Urban greening to cool towns and cities: A systematic review of the empirical evidence. Landscape and Urban Planning, 97(3), 147-155.
[40] Jabareen, Y. R. (2006). Sustainable urban forms: their typologies, models, and concepts. Journal of Planning Education and Research, 26(1), 38-52.
[41] Sharifi, A., & Murayama, Y. (2013). A critical review of seven dimensions of urban sustainability assessment: Towards developing a tool for measuring urban sustainability. Environmental Impact Assessment Review, 38, 73-87.
[42] Handy, S. L., Boarnet, M. G., Ewing, R., & Killingsworth, R. E. (2002). How the built environment affects physical activity: views from urban planning. American Journal of Preventive Medicine, 23(2), 64-73.
[43] Wong, T. H. F. (2006). Water sensitive urban design – the state of play. Water Science & Technology, 53(3), 1-18.
[44] Crawley, D. B., Roth, W. J., Briggs, R. S., Fischer, D. E., Hall, J. Q., & Torcellini, P. A. (2001). Contrasting the effects of detailed and simplified building energy modeling on energy efficiency policy development. Energy Policy, 29(11), 979-990.
[45] Blumstein, C., Kreig, B., Schipper, L., & York, C. (1980). Overcoming social and institutional barriers to energy conservation. Energy, 5(4), 355-371.
[46] Ebi, K. L., & Meehl, G. A. (2007). The heat is on: climate change and heat waves. Wiley Interdisciplinary Reviews: Climate Change, 1(1), 91-118.
[47] Bernard, S. M., & McGeehin, M. A. (2004). Climate change and human health. Emerging Infectious Diseases, 10(5), 585.
[48] Preston, B. L., & Stafford-Smith, M. (2009). Framing vulnerability and adaptive capacity assessment: Discussion. Wiley Interdisciplinary Reviews: Climate Change, 1(1), 147-162.
[49] Dovers, S., & Hezri, A. A. (2010). Institutions and policy processes for climate change adaptation in developed countries. Wiley Interdisciplinary Reviews: Climate Change, 1(2), 235-253.
[50] Hallegatte, S. (2009). Strategies to adapt to an uncertain climate. Global Environmental Change, 19(2), 240-247.
[51] Dessai, S., Hulme, M., Lempert, R., & Pielke Jr, R. (2009). Climate prediction: When policy makers ask ‘how much confidence do you have?’ Philosophical Transactions of the Royal Society A: Mathematical, Physical and Engineering Sciences, 367(1895), 1511-1522.
[52] Moomaw, W. R., & Wormser, A. (2011). Energy policy and climate change: Bridging the gaps. Island Press.
[53] Alberti, M. (2008). Advances in urban ecology: integrating humans and ecological processes in urban ecosystems. Springer Science & Business Media.
[54] Wilkinson, S., & Reed, R. (2008). Sustainable construction. John Wiley & Sons.
[55] Berke, P. R. (2002). Does sustainable development offer a new direction for planning? Challenges for the 21st century. Journal of Planning Literature, 17(1), 21-36.
[56] Stern, N. (2007). The economics of climate change: the Stern review. Cambridge University Press.
[57] Lovins, A. B. (2011). Reinventing fire: Bold business solutions for the new energy era. Chelsea Green Publishing.
[58] Sovacool, B. K. (2009). Evaluating what future energy policies are “best”: towards a more policy-relevant multi-criteria assessment of the alternatives. Energy Policy, 37(10), 3824-3835.
[59] Agrawala, S., & Fankhauser, S. (2008). Economic aspects of adaptation to climate change: costs, benefits and policy instruments. OECD Publishing.
[60] Barrett, S. (2003). Environment and statecraft: the strategy of environmental treaty-making. Oxford University Press.
This report highlights a critical need for proactive measures, especially considering the disproportionate vulnerability of certain populations like the elderly and low-income households. Exploring community-based initiatives that provide targeted support during heatwaves could significantly enhance resilience and safeguard public health.
Thank you for your insightful comment! I agree that community-based initiatives are crucial. Exploring programs like local cooling centers, neighborhood support networks, and accessible transportation during heatwaves could greatly enhance resilience, especially for our elderly and low-income neighbors. Let’s discuss effective implementation strategies!
Editor: FocusNews.Uk
Thank you to our Sponsor Focus 360 Energy
The report rightly emphasizes building design and retrofitting. Could lifecycle assessments of building materials further inform decisions, considering both embodied carbon and long-term thermal performance to ensure truly sustainable adaptation strategies?
That’s a great point! Incorporating lifecycle assessments would definitely provide a more comprehensive understanding of the true environmental impact. Accounting for embodied carbon along with thermal performance ensures we’re not just shifting the problem elsewhere. This holistic approach is key to truly sustainable building practices. Thank you for raising it!
Editor: FocusNews.Uk
Thank you to our Sponsor Focus 360 Energy
This report rightly points out the importance of considering urban planning. Prioritizing green infrastructure and mixed-use development to improve ventilation and reduce emissions seem like key strategies for mitigating the impact of increasing temperatures in urban environments.
Thanks for highlighting the importance of urban planning! Expanding on that, integrating smart technologies like real-time environmental monitoring and adaptive building controls could further optimize resource use and enhance the effectiveness of green infrastructure in mitigating urban heat. What are your thoughts on this potential integration?
Editor: FocusNews.Uk
Thank you to our Sponsor Focus 360 Energy
This report is so thorough, I’m now convinced my thermostat is plotting against me. Seriously though, integrating behavioural adjustments, like siesta adoption, alongside tech and policy, could be the surprisingly effective ‘cooling hack’ we’ve been missing. Think global, act local…ly asleep mid-afternoon.
That’s a fantastic point! The idea of incorporating behavioral shifts like siestas, alongside tech and policy, is definitely worth exploring. It’s interesting to consider how cultural norms can evolve to enhance climate resilience. How might businesses adapt to accommodate such shifts in work patterns for optimal productivity and well-being?
Editor: FocusNews.Uk
Thank you to our Sponsor Focus 360 Energy
This report effectively highlights the need for adaptation strategies. Further research into cost-effective, scalable solutions for retrofitting existing building stock, particularly in vulnerable communities, would be incredibly valuable for practical implementation and wider adoption.