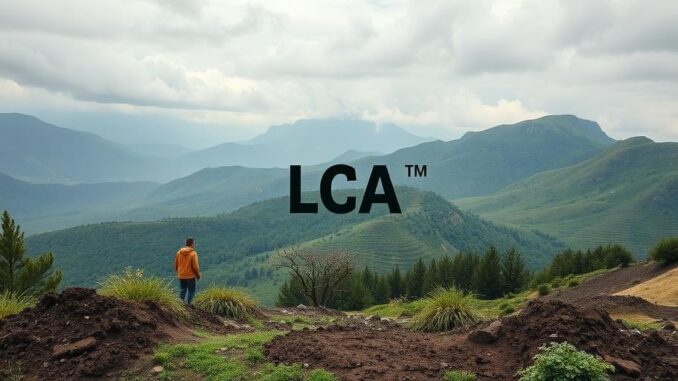
Abstract
Life Cycle Assessment (LCA) has emerged as a crucial methodology for evaluating the environmental impacts associated with a product, process, or service throughout its entire lifespan, from raw material extraction to end-of-life management. This research report delves into the framework of LCA, focusing on its evolution and adaptation within the context of a circular economy. It explores not only the established methodologies (ISO 14040 and ISO 14044) but also emerging advancements in data acquisition, impact assessment methods, and the integration of socio-economic factors. A critical analysis of the challenges surrounding data quality, uncertainty, and the complexities of modeling circularity is presented. Furthermore, the report examines the role of LCA in driving innovation, informing policy decisions, and fostering sustainable practices across diverse industries. It concludes by outlining future research directions necessary to enhance the applicability and effectiveness of LCA in a rapidly evolving global landscape, particularly in the transition towards a more resource-efficient and resilient circular economy.
Many thanks to our sponsor Focus 360 Energy who helped us prepare this research report.
1. Introduction
The accelerating depletion of natural resources and the escalating impacts of climate change have spurred a global movement towards sustainability. At the heart of this movement lies the imperative to understand and mitigate the environmental burdens associated with our consumption patterns. Life Cycle Assessment (LCA) provides a comprehensive framework for quantifying these burdens across the entire value chain, from “cradle to grave.” Developed in the late 1960s and 1970s, LCA has since matured into a standardized methodology, guided by the ISO 14040 and ISO 14044 standards [1, 2]. These standards delineate the principles, framework, requirements, and guidelines for conducting and interpreting LCA studies.
However, the context in which LCA is applied is rapidly changing. The linear “take-make-dispose” model is increasingly recognized as unsustainable, prompting a shift towards a circular economy. A circular economy aims to minimize waste and pollution, keep products and materials in use for longer, and regenerate natural systems [3]. This paradigm shift presents both opportunities and challenges for LCA. While LCA can be instrumental in identifying the environmental benefits of circular strategies, such as reuse, recycling, and remanufacturing, it also requires adaptations to accurately model the complexities of closed-loop systems and the potential trade-offs involved. Therefore, this research report aims to provide a comprehensive overview of LCA, examining its evolution, challenges, and future directions within the evolving landscape of a circular economy.
Many thanks to our sponsor Focus 360 Energy who helped us prepare this research report.
2. Framework of Life Cycle Assessment
The ISO 14040 and 14044 standards define the four key phases of an LCA study:
-
2.1 Goal and Scope Definition: This initial phase sets the stage for the entire study. The goal defines the intended application and audience of the LCA. The scope defines the product system under investigation, its function, functional unit (the quantified performance of a product system for use as a reference unit), system boundary (which processes are included), allocation rules (how environmental burdens are assigned to different products in multi-output processes), and impact categories to be assessed. Clearly defining the goal and scope is crucial for ensuring the relevance, reliability, and comparability of the LCA results. Careful consideration should be given to defining a functional unit that is representative and allows for meaningful comparisons between different product systems [4]. For instance, comparing the environmental impact of two different light bulbs requires a functional unit that specifies the desired illuminance (e.g., lumens) and lifespan (e.g., hours). The system boundary should be carefully chosen to include all significant processes while excluding minor processes that would not significantly alter the results. For example, the system boundary for an LCA of a smartphone might include raw material extraction, manufacturing, transportation, use phase (including electricity consumption), and end-of-life management, but it might exclude the environmental impact of the packaging used to ship individual components to the factory if these are deemed insignificant relative to other processes.
-
2.2 Life Cycle Inventory (LCI) Analysis: The LCI phase involves collecting and quantifying data on all relevant inputs (e.g., raw materials, energy) and outputs (e.g., emissions to air, water, and soil, waste) associated with each process within the system boundary. This is often the most time-consuming and data-intensive phase of the LCA. LCI data can be obtained from various sources, including databases (e.g., Ecoinvent, GaBi), literature, industry reports, and primary data collection from companies. The quality and availability of LCI data are critical factors influencing the accuracy and reliability of the LCA results. Addressing data gaps and uncertainties is a significant challenge, often requiring the use of assumptions and modeling techniques. The use of foreground and background data are also differentiated in LCI: foreground data pertains to the specific production processes of the product under study (e.g., data gathered directly from a manufacturer), whereas background data pertains to the upstream (e.g., electricity production, chemical manufacturing) processes needed to deliver goods or services to the foreground system [5].
-
2.3 Life Cycle Impact Assessment (LCIA): The LCIA phase translates the LCI data into environmental impact scores for various impact categories, such as climate change, ozone depletion, acidification, eutrophication, resource depletion, and human toxicity. LCIA methods use characterization factors to convert LCI flows into impact potentials. For example, emissions of different greenhouse gases are multiplied by their respective global warming potentials (GWP) to obtain a climate change impact score. Several LCIA methods are available, each with its own strengths and weaknesses, and the choice of method can significantly influence the LCA results [6]. Common LCIA methods include ReCiPe, CML, and TRACI. Different methods employ different midpoint and endpoint indicators, as well as differing normalization schemes. Midpoint indicators are at a relatively early stage in the cause-effect chain (e.g., global warming potential), while endpoint indicators reflect damages to areas of protection, such as human health, ecosystems, and resources. The choice of LCIA method should be justified based on the goal and scope of the study and the specific regional context.
-
2.4 Interpretation: The interpretation phase involves analyzing the LCA results, identifying significant environmental hotspots, evaluating the robustness of the results, and drawing conclusions and recommendations. Sensitivity analysis and uncertainty analysis are essential components of the interpretation phase. Sensitivity analysis assesses the influence of changes in input data or assumptions on the LCA results. Uncertainty analysis quantifies the overall uncertainty associated with the LCA results, taking into account uncertainties in the LCI data, LCIA methods, and modeling assumptions. The interpretation phase should also consider the limitations of the LCA methodology and the potential trade-offs between different environmental impacts. For example, reducing greenhouse gas emissions might lead to an increase in water consumption or resource depletion. The ultimate goal of the interpretation phase is to provide actionable insights for decision-makers to improve the environmental performance of products, processes, and services.
Many thanks to our sponsor Focus 360 Energy who helped us prepare this research report.
3. Advancements in LCA Methodologies
While the core framework of LCA remains grounded in the ISO 14040 and 14044 standards, ongoing research and development have led to significant advancements in various aspects of the methodology:
-
3.1 Data Acquisition and Management: The availability and quality of LCI data are crucial for conducting reliable LCA studies. Advancements in data acquisition and management include the development of more comprehensive and transparent LCI databases, the use of machine learning techniques to fill data gaps, and the integration of blockchain technology to improve data traceability and security [7]. Furthermore, the rise of Big Data and the Internet of Things (IoT) provides new opportunities for collecting real-time data on resource consumption and emissions, enabling more accurate and dynamic LCAs. The development of standardized data formats and protocols facilitates the exchange of LCI data between different organizations and software tools.
-
3.2 Impact Assessment Methods: Traditional LCIA methods primarily focus on environmental impacts. However, there is a growing recognition of the need to integrate socio-economic factors into LCA. Social Life Cycle Assessment (S-LCA) is a complementary methodology that assesses the social impacts associated with a product’s life cycle, such as labor rights, health and safety, and community impacts [8]. Integrating environmental and social impacts into a single framework remains a challenge, but progress is being made in developing multi-criteria decision analysis (MCDA) tools and integrated sustainability assessments. Furthermore, advancements in environmental impact assessment methods are addressing emerging environmental concerns, such as microplastic pollution, biodiversity loss, and land use change.
-
3.3 Modeling Circularity: The transition to a circular economy requires LCA to accurately model the complexities of closed-loop systems. This includes accounting for the benefits of reuse, recycling, and remanufacturing, as well as the potential trade-offs involved. Dynamic LCA, which considers the time-dependent nature of environmental impacts, is particularly relevant for assessing the long-term benefits of circular strategies. For example, the climate change impact of recycling aluminum depends on the displacement of primary aluminum production, which in turn depends on the future electricity mix and the efficiency of recycling processes. Circularity metrics, such as material circularity indicator (MCI), are being developed to quantify the circularity performance of products and processes, providing a useful complement to LCA [9]. These metrics allow for a direct comparison of the degree of circularity achieved by different products and services. However, they should be used in conjunction with LCA to ensure that circular strategies do not inadvertently shift environmental burdens to other parts of the life cycle.
Many thanks to our sponsor Focus 360 Energy who helped us prepare this research report.
4. Challenges and Limitations of LCA
Despite its widespread adoption and ongoing advancements, LCA still faces several challenges and limitations:
-
4.1 Data Quality and Uncertainty: The accuracy and reliability of LCA results depend heavily on the quality of the LCI data. Data gaps, uncertainties, and variability in LCI data can significantly influence the LCA results. Addressing data quality issues requires rigorous data validation, sensitivity analysis, and uncertainty analysis. The use of proxy data and modeling assumptions should be transparently documented and justified. Furthermore, the inherent uncertainty associated with LCIA methods and characterization factors should be acknowledged and addressed. Monte Carlo simulation is a common technique for propagating uncertainties through the LCA model and quantifying the overall uncertainty in the results [10].
-
4.2 System Boundary Definition: Defining the system boundary is a critical and often subjective decision that can significantly influence the LCA results. Narrow system boundaries may underestimate the true environmental impacts, while overly broad system boundaries can make the LCA computationally complex and data-intensive. The system boundary should be carefully chosen to include all significant processes while excluding minor processes that would not significantly alter the results. The cut-off criteria used to exclude processes from the system boundary should be clearly documented and justified.
-
4.3 Allocation Issues: Allocation is required when a process produces multiple products or services. Assigning environmental burdens to different products in multi-output processes can be challenging and subjective. Several allocation methods are available, including physical allocation (based on mass or energy content), economic allocation (based on market value), and system expansion (avoiding allocation by expanding the system boundary). The choice of allocation method can significantly influence the LCA results. The ISO 14044 standard provides guidance on allocation procedures, but the selection of the most appropriate method often requires careful consideration of the specific context and the goal of the study [2].
-
4.4 Modeling Complexity: LCA models can become very complex, particularly when dealing with complex product systems and circular economy scenarios. Modeling the interactions between different processes and the feedback loops in circular systems requires advanced modeling techniques. Simplifications and assumptions are often necessary to make the LCA computationally tractable, but these simplifications can introduce errors and uncertainties. The use of process-based LCA, which models individual processes in detail, can be computationally intensive for complex product systems. Hybrid LCA methods, which combine process-based LCA with economic input-output analysis, can provide a more efficient way to model complex systems [11].
-
4.5 Interpretation and Communication: Interpreting and communicating LCA results effectively is crucial for informing decision-makers and promoting sustainable practices. LCA results are often complex and can be difficult to understand for non-experts. The use of clear and concise visualizations and narratives can help to communicate LCA results effectively. Furthermore, it is important to acknowledge the limitations of the LCA methodology and the uncertainties associated with the results. Comparing LCA results from different studies can be challenging due to differences in scope, data, and methods. Standardized reporting formats and guidelines can help to improve the comparability of LCA results.
Many thanks to our sponsor Focus 360 Energy who helped us prepare this research report.
5. The Role of LCA in Driving Innovation and Sustainable Practices
Despite its challenges, LCA plays a crucial role in driving innovation and promoting sustainable practices across diverse industries:
-
5.1 Product Design and Development: LCA can be used to identify environmental hotspots in the product life cycle and to inform design decisions that reduce environmental impacts. Eco-design principles, such as material selection, design for durability, design for disassembly, and design for recyclability, can be integrated into the product development process using LCA as a guiding tool. For example, LCA can be used to compare the environmental impacts of different materials, manufacturing processes, and packaging options. By considering the entire life cycle, LCA can help to avoid shifting environmental burdens from one stage of the life cycle to another.
-
5.2 Process Optimization: LCA can be used to identify opportunities for improving the environmental performance of industrial processes. By analyzing the inputs and outputs of each process, LCA can help to identify areas where resource consumption and emissions can be reduced. Process optimization can involve changes in raw material inputs, energy efficiency improvements, waste reduction, and the adoption of cleaner production technologies. LCA can also be used to evaluate the environmental benefits of alternative process configurations.
-
5.3 Policy Development: LCA can inform policy decisions related to environmental regulations, product standards, and sustainable consumption. Life cycle thinking is increasingly being incorporated into policy frameworks, such as extended producer responsibility (EPR) schemes, eco-labeling programs, and carbon footprinting initiatives. LCA can provide a scientific basis for setting environmental targets and for evaluating the effectiveness of policy interventions. For example, LCA can be used to assess the environmental impacts of different waste management options, such as landfilling, incineration, and recycling, and to inform policies that promote the most sustainable waste management practices.
-
5.4 Investment Decisions: Investors are increasingly considering environmental, social, and governance (ESG) factors in their investment decisions. LCA can provide valuable information for assessing the environmental performance of companies and products. By quantifying the environmental risks and opportunities associated with different investments, LCA can help investors to make more informed decisions that support sustainable development. Furthermore, LCA can be used to evaluate the environmental benefits of green technologies and sustainable business models.
Many thanks to our sponsor Focus 360 Energy who helped us prepare this research report.
6. Future Directions
To further enhance the applicability and effectiveness of LCA in a rapidly evolving global landscape, several research directions are needed:
-
6.1 Improving Data Availability and Quality: Continued efforts are needed to improve the availability and quality of LCI data. This includes the development of more comprehensive and transparent LCI databases, the use of advanced data analytics techniques to fill data gaps, and the integration of blockchain technology to improve data traceability and security. Furthermore, research is needed to develop standardized data formats and protocols to facilitate the exchange of LCI data between different organizations and software tools.
-
6.2 Enhancing Impact Assessment Methods: Further research is needed to enhance impact assessment methods to address emerging environmental concerns, such as microplastic pollution, biodiversity loss, and land use change. Integrated assessment frameworks that combine environmental, social, and economic impacts are also needed. Developing robust methods for valuing ecosystem services and incorporating them into LCA is a significant challenge. Furthermore, research is needed to develop spatially explicit LCIA methods that account for the location-specific impacts of environmental emissions.
-
6.3 Modeling Dynamic and Complex Systems: Advanced modeling techniques are needed to accurately model dynamic and complex systems, such as circular economy systems and urban systems. This includes the development of dynamic LCA models that consider the time-dependent nature of environmental impacts and the use of agent-based modeling techniques to simulate the interactions between different actors in the system. Furthermore, research is needed to develop hybrid LCA methods that combine process-based LCA with economic input-output analysis and other modeling techniques.
-
6.4 Integrating Uncertainty and Variability: Robust methods are needed to quantify and manage uncertainty and variability in LCA. This includes the use of Monte Carlo simulation, Bayesian analysis, and other statistical techniques. Furthermore, research is needed to develop methods for communicating uncertainty to decision-makers in a clear and understandable way. The use of scenario analysis can help to explore the potential range of outcomes under different assumptions.
-
6.5 Developing User-Friendly Tools and Guidelines: User-friendly tools and guidelines are needed to make LCA more accessible to a wider audience. This includes the development of online LCA software tools, training programs, and case studies. Furthermore, research is needed to develop simplified LCA methods for specific applications, such as product carbon footprinting and building energy analysis. The development of standardized reporting formats and guidelines can help to improve the comparability of LCA results.
Many thanks to our sponsor Focus 360 Energy who helped us prepare this research report.
7. Conclusion
Life Cycle Assessment has evolved into a powerful tool for understanding and mitigating the environmental impacts of products, processes, and services. Its comprehensive framework, encompassing goal and scope definition, life cycle inventory, life cycle impact assessment, and interpretation, provides a systematic approach to evaluating environmental burdens across the entire value chain. As the world transitions towards a circular economy, LCA plays an increasingly important role in identifying the environmental benefits of circular strategies and informing sustainable decision-making. While challenges remain in terms of data quality, modeling complexity, and communication, ongoing advancements in data acquisition, impact assessment methods, and modeling techniques are expanding the applicability and effectiveness of LCA. By continuing to invest in research and development, and by promoting the widespread adoption of LCA principles, we can harness its potential to drive innovation, inform policy, and foster a more sustainable future.
Many thanks to our sponsor Focus 360 Energy who helped us prepare this research report.
References
[1] ISO 14040:2006. Environmental management — Life cycle assessment — Principles and framework. International Organization for Standardization, Geneva.
[2] ISO 14044:2006. Environmental management — Life cycle assessment — Requirements and guidelines. International Organization for Standardization, Geneva.
[3] Ellen MacArthur Foundation. (2015). Towards a circular economy: Business rationale for an accelerated transition.
[4] Finnveden, G., Hauschild, M. Z., Ekvall, T., Guinée, J., Heijungs, R., Hellweg, S., … & Rebitzer, G. (2009). Recent developments in life cycle assessment. Journal of environmental management, 91(1), 1-21.
[5] Weidema, B. P., Wesnæs, M. S., Hermansen, J., Kristensen, T., Halberg, N., Eder, P., & Delgado, L. (2008). Environmental improvement potentials of agriculture. Journal of environmental management, 86(4), 683-695.
[6] Jolliet, O., Antón, A., Arenas, E., Boulay, A. M., Deshayes, M., Frischknecht, R., … & Vigon, B. (2018). Global guidance for life cycle impact assessment indicators: key scientific recommendations. The International Journal of Life Cycle Assessment, 23(6), 1137-1146.
[7] Simão, A., Ribeiro, I., Dias, A. C., & Bernardes, A. M. (2021). Blockchain for sustainability in supply chains: Literature review and future research directions. Resources, Conservation and Recycling, 169, 105510.
[8] Benoît-Norris, C., Traverso, M., & Wenzel, H. (2011). Applying social life cycle assessment: towards more sustainable decisions. Springer Science & Business Media.
[9] Saidani, M., Yannou, B., Leroy, Y., Cluzel, F., & Kendall, A. (2019). How to assess product circularity? A review of methods. Journal of Cleaner Production, 207, 570-588.
[10] Heijungs, R., & Huijbregts, M. A. (2004). A review of methods to quantify uncertainty in life cycle assessment. The International Journal of Life Cycle Assessment, 9(2), 101-112.
[11] Suh, S., & Huppes, G. (2005). Methods for life cycle inventory of a product. Journal of cleaner production, 13(7), 687-697.
The report highlights the challenges of data quality in LCA. Given the increasing reliance on secondary data sources and modelling to fill data gaps, what strategies can be employed to enhance transparency and validation of these data inputs, ensuring the reliability of LCA results?