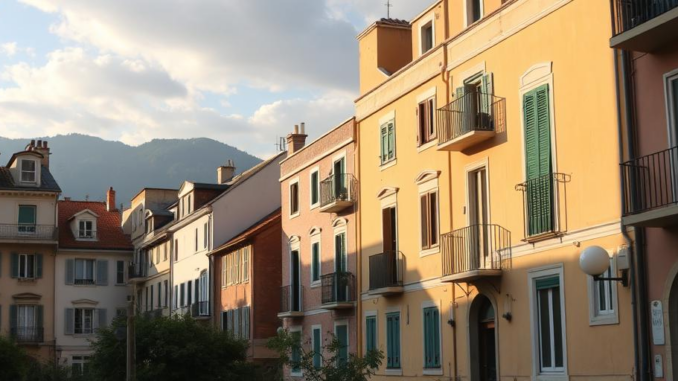
Abstract
This research report investigates the critical role of climate in the design and implementation of effective solar shading strategies for buildings. Recognizing that the optimal shading solution is intrinsically linked to the specific climatic conditions of a region, this study conducts a comprehensive analysis across diverse climatic zones, including arid, tropical, temperate, and cold climates. The report delves into the complex interplay between solar radiation, temperature fluctuations, humidity levels, and prevailing wind patterns, and how these factors influence the performance of various shading systems. A key focus is on the selection of appropriate shading materials, designs, and control strategies tailored to each climatic context. Furthermore, the report explores advanced modeling and simulation techniques for predicting shading performance and optimizing energy efficiency. The findings highlight the significance of a climate-responsive design approach to solar shading, leading to improved occupant comfort, reduced energy consumption, and enhanced building sustainability. The report also addresses the challenges and opportunities associated with implementing innovative shading technologies, such as dynamic shading systems and phase change materials, in different climates.
Many thanks to our sponsor Focus 360 Energy who helped us prepare this research report.
1. Introduction
The imperative to reduce energy consumption in buildings has spurred significant advancements in building design and technology. Among the various passive design strategies, solar shading stands out as a crucial element in mitigating solar heat gain, particularly in regions with high solar irradiance. Solar shading encompasses a range of architectural features and mechanical systems designed to block direct sunlight from entering a building, thereby reducing cooling loads and improving thermal comfort. The effectiveness of any solar shading solution is inextricably linked to the prevailing climatic conditions. A design that performs optimally in a temperate climate may be entirely unsuitable for an arid or tropical environment. Therefore, a nuanced understanding of the climate’s impact on solar shading is essential for achieving optimal building performance.
This report aims to provide a comprehensive overview of solar shading strategies across diverse climatic zones. It explores the specific challenges and opportunities presented by each climate and examines the performance of various shading systems under varying weather conditions. Furthermore, the report delves into advanced modeling and simulation techniques for predicting shading performance and optimizing energy efficiency.
Many thanks to our sponsor Focus 360 Energy who helped us prepare this research report.
2. Climatic Zones and Their Impact on Solar Shading
The Earth’s diverse climates can be broadly categorized into several zones, each characterized by unique combinations of temperature, humidity, precipitation, and solar radiation. Understanding these climatic variations is crucial for designing effective solar shading strategies.
2.1 Arid Climates
Arid climates, characterized by high temperatures, low humidity, and intense solar radiation, present a significant challenge for building design. The primary goal of solar shading in arid climates is to minimize solar heat gain and reduce cooling loads. Deep overhangs, recessed windows, and external shading devices are commonly employed to block direct sunlight from entering the building envelope. Materials with high reflectivity and low thermal conductivity are preferred to minimize heat absorption. Evaporative cooling techniques, such as vegetation and water features, can also be integrated with solar shading to enhance cooling performance. It is also important to consider dust accumulation which will affect the performance of the shading solution over time.
2.2 Tropical Climates
Tropical climates are characterized by high temperatures, high humidity, and abundant rainfall. Solar shading in tropical climates must address both solar heat gain and the need for natural ventilation. Overhangs and louvers are commonly used to block direct sunlight while allowing airflow. Shading devices should be designed to withstand heavy rainfall and prevent water accumulation. The selection of durable, moisture-resistant materials is essential to ensure long-term performance. Biophilic designs incorporating vegetation can provide natural shading and enhance the aesthetic appeal of buildings. The risk of mould growth is a primary concern in selecting appropriate materials.
2.3 Temperate Climates
Temperate climates experience distinct seasons with moderate temperatures and humidity levels. Solar shading strategies in temperate climates must balance the need for solar heat gain in winter with the need for solar heat rejection in summer. Adjustable shading devices, such as blinds and shutters, allow occupants to control the amount of sunlight entering the building. Deciduous trees can provide seasonal shading, blocking sunlight in summer while allowing it to penetrate in winter. The orientation of the building is critical to maximise solar gain in winter while minimising it in summer.
2.4 Cold Climates
Cold climates are characterized by low temperatures, long winters, and limited solar radiation. The primary goal of solar shading in cold climates is to maximize solar heat gain during the winter months to reduce heating loads. South-facing windows and transparent shading devices, such as clear glazing, allow sunlight to enter the building and provide passive solar heating. Overhangs and other shading devices should be designed to minimize shading during the winter months. Thermal mass materials can be used to store solar heat and release it gradually, improving thermal comfort and reducing energy consumption. The design and positioning of shading solutions must consider snow accumulation.
Many thanks to our sponsor Focus 360 Energy who helped us prepare this research report.
3. Solar Shading Design Considerations
Designing effective solar shading systems requires careful consideration of several factors, including solar geometry, building orientation, shading device geometry, and material properties.
3.1 Solar Geometry and Orientation
The sun’s position in the sky varies throughout the day and year, depending on latitude and time of year. Understanding solar geometry is crucial for determining the optimal orientation and configuration of solar shading devices. Building orientation plays a significant role in determining the amount of solar radiation that strikes the building envelope. In general, east- and west-facing facades receive the most intense solar radiation during the morning and afternoon, respectively, while south-facing facades receive the most solar radiation during the winter months. The height of adjacent buildings or terrain can have a significant effect on the amount of solar radiation reaching the building. Computer simulations can be used to accurately model solar geometry and predict shading performance.
3.2 Shading Device Geometry
The geometry of solar shading devices, including their size, shape, and spacing, significantly affects their performance. Overhangs, for example, can effectively block direct sunlight from entering the building during the summer months, while allowing sunlight to penetrate during the winter months. Louvers can be oriented horizontally or vertically to provide shading from different angles. The spacing between louvers affects the amount of sunlight that is blocked. The design of the shading device must consider the specific climatic conditions and building orientation. The shape of the shading solution also plays a role in determining how well it can disperse incoming sunlight and the shading pattern produced.
3.3 Material Properties
The material properties of solar shading devices, including their reflectivity, absorptivity, and thermal conductivity, affect their ability to block solar heat. Materials with high reflectivity and low thermal conductivity are preferred to minimize heat absorption. The color of the shading device also affects its performance, with lighter colors reflecting more sunlight than darker colors. The durability and maintenance requirements of the material should also be considered, particularly in harsh climates. The material’s thermal mass can contribute to its overall thermal performance. The long-term weathering and degradation of the material under specific climatic conditions should be assessed.
3.4 Control Strategies
The control strategy for solar shading devices can significantly affect their performance. Fixed shading devices provide constant shading, while adjustable shading devices allow occupants to control the amount of sunlight entering the building. Automated control systems can adjust shading devices based on real-time weather conditions and occupancy patterns. Sensors can be used to detect solar radiation, temperature, and occupancy, and the control system can adjust the shading devices accordingly. Control strategies can be optimized to minimize energy consumption and improve thermal comfort. An intelligent control system can also take into account the angle of the sun and adjust the shading dynamically.
Many thanks to our sponsor Focus 360 Energy who helped us prepare this research report.
4. Solar Shading Technologies
Several solar shading technologies are available, each with its advantages and disadvantages. These technologies can be broadly categorized into fixed shading devices, adjustable shading devices, and advanced shading systems.
4.1 Fixed Shading Devices
Fixed shading devices are permanently installed and provide constant shading. Common examples include overhangs, fins, and louvers. Fixed shading devices are relatively inexpensive and require minimal maintenance. However, they offer limited flexibility and may not be suitable for all climates. Fixed shading is relatively insensitive to human error and equipment failure.
4.2 Adjustable Shading Devices
Adjustable shading devices allow occupants to control the amount of sunlight entering the building. Common examples include blinds, shutters, and curtains. Adjustable shading devices offer greater flexibility than fixed shading devices, allowing occupants to adjust the shading based on their preferences and needs. However, they require more maintenance and may be more expensive than fixed shading devices. The effectiveness of adjustable shading is dependent on the user adopting the most suitable configuration.
4.3 Advanced Shading Systems
Advanced shading systems incorporate innovative technologies to enhance shading performance and energy efficiency. Examples include dynamic shading systems, electrochromic glazing, and phase change materials.
4.3.1 Dynamic Shading Systems
Dynamic shading systems automatically adjust their position based on real-time weather conditions and occupancy patterns. These systems can be controlled by sensors that detect solar radiation, temperature, and occupancy. Dynamic shading systems offer optimal shading performance and energy efficiency. However, they are more expensive than fixed or adjustable shading devices and require sophisticated control systems. These systems can respond to changing sunlight angles throughout the day.
4.3.2 Electrochromic Glazing
Electrochromic glazing changes its transparency in response to an applied voltage. This allows occupants to control the amount of sunlight entering the building. Electrochromic glazing can reduce solar heat gain and improve thermal comfort. However, it is more expensive than conventional glazing and requires an electrical power source. The switching speed can be a limiting factor for fast changing sunlight levels.
4.3.3 Phase Change Materials
Phase change materials (PCMs) absorb and release heat as they change state (e.g., from solid to liquid). PCMs can be incorporated into solar shading devices to reduce temperature fluctuations and improve thermal comfort. They can store excess heat during the day and release it at night. The incorporation of PCMs can provide better insulation.
Many thanks to our sponsor Focus 360 Energy who helped us prepare this research report.
5. Modeling and Simulation Techniques
Modeling and simulation techniques are essential for predicting the performance of solar shading systems and optimizing their design. Several software tools are available for simulating solar radiation, heat transfer, and building energy performance.
5.1 Radiance
Radiance is a widely used software tool for simulating lighting and daylighting. It can accurately model solar radiation and predict shading performance. Radiance can be used to evaluate the impact of different shading strategies on indoor illuminance levels and visual comfort. It uses backward ray tracing techniques to determine the amount of light arriving at specific points in a space.
5.2 EnergyPlus
EnergyPlus is a comprehensive building energy simulation program developed by the U.S. Department of Energy. It can simulate heat transfer, ventilation, and air conditioning systems. EnergyPlus can be used to evaluate the impact of solar shading on building energy consumption and thermal comfort. It integrates with other simulation tools, such as Radiance, to provide a comprehensive analysis of building performance. Detailed weather data is required to achieve accurate results.
5.3 Computational Fluid Dynamics (CFD)
Computational Fluid Dynamics (CFD) is a powerful tool for simulating airflow and heat transfer. CFD can be used to analyze the impact of solar shading on natural ventilation and thermal comfort. It can predict air temperature, velocity, and humidity distributions within a building. CFD simulations can help optimize the design of shading devices to maximize natural ventilation and minimize solar heat gain. The complexity of CFD simulations requires significant computational resources.
Many thanks to our sponsor Focus 360 Energy who helped us prepare this research report.
6. Case Studies
This section presents case studies of buildings that have successfully implemented solar shading strategies in different climatic zones.
6.1 Arid Climate: Masdar Institute, Abu Dhabi
The Masdar Institute in Abu Dhabi incorporates several solar shading strategies to minimize solar heat gain and reduce cooling loads. The buildings feature deep overhangs, recessed windows, and external shading devices. The materials used have high reflectivity and low thermal conductivity. The campus also utilizes evaporative cooling techniques to enhance cooling performance. The design is an example of an integrated approach to sustainable building design in a hot, arid climate. The specific location of the building with respect to surrounding buildings was carefully considered.
6.2 Tropical Climate: Gardens by the Bay, Singapore
Gardens by the Bay in Singapore features a series of Supertrees that provide shading and vertical planting. The Supertrees are covered in vegetation and equipped with solar panels. They provide shading for visitors and reduce the heat island effect. The design is an example of biophilic architecture and sustainable urban design in a tropical climate. The ability of the design to withstand storm conditions was an important design consideration.
6.3 Temperate Climate: Bullitt Center, Seattle
The Bullitt Center in Seattle incorporates several solar shading strategies to balance solar heat gain and rejection. The building features adjustable shading devices and deciduous trees that provide seasonal shading. The orientation of the building maximizes solar gain in winter while minimizing it in summer. The Bullitt Center is considered one of the greenest commercial buildings in the world. Its design focuses on energy efficiency and water conservation.
Many thanks to our sponsor Focus 360 Energy who helped us prepare this research report.
7. Challenges and Opportunities
Implementing effective solar shading strategies presents several challenges, including cost, complexity, and maintenance. However, it also offers significant opportunities for improving building performance, reducing energy consumption, and enhancing occupant comfort.
7.1 Cost
The initial cost of solar shading systems can be a barrier to their implementation. However, the long-term energy savings can often offset the initial cost. Government incentives and tax credits can also help reduce the cost of solar shading systems. Life cycle cost analysis is essential for evaluating the economic viability of solar shading projects. The maintenance costs should also be included in this analysis.
7.2 Complexity
Designing and implementing effective solar shading systems can be complex, requiring expertise in solar geometry, building physics, and control systems. Integrated design teams that include architects, engineers, and energy consultants are essential for successful solar shading projects. Advanced modeling and simulation tools can help simplify the design process. The commissioning process is also important to ensure that the shading system is operating correctly.
7.3 Maintenance
Solar shading systems require regular maintenance to ensure their optimal performance. Maintenance activities include cleaning, repairing, and replacing components. The maintenance requirements of solar shading systems should be considered during the design phase. Durable and low-maintenance materials should be selected. Automated maintenance systems can reduce the need for manual intervention. A maintenance plan is vital to ensure the optimal performance of the system.
7.4 Opportunities
Solar shading offers significant opportunities for improving building performance, reducing energy consumption, and enhancing occupant comfort. It can reduce cooling loads, improve thermal comfort, and provide natural daylighting. Solar shading can also enhance the aesthetic appeal of buildings and contribute to sustainable urban development. It also reduces glare which improves comfort.
Many thanks to our sponsor Focus 360 Energy who helped us prepare this research report.
8. Future Trends
Several future trends are expected to shape the development of solar shading technologies, including the integration of smart materials, advanced control systems, and building-integrated photovoltaics.
8.1 Smart Materials
Smart materials, such as electrochromic glazing and thermochromic materials, can dynamically adjust their properties in response to changing environmental conditions. These materials can automatically adjust the amount of sunlight entering the building, improving energy efficiency and thermal comfort. Self cleaning materials are expected to become more prevalent in solar shading applications.
8.2 Advanced Control Systems
Advanced control systems can optimize the performance of solar shading systems based on real-time weather conditions, occupancy patterns, and energy prices. These systems can learn from past performance and adapt to changing conditions. Artificial intelligence and machine learning techniques are expected to play an increasing role in controlling solar shading systems. IoT (internet of things) sensors will provide more granular and accurate information. The challenge is to ensure the system is robust and resistant to cyber attacks.
8.3 Building-Integrated Photovoltaics (BIPV)
Building-integrated photovoltaics (BIPV) integrate solar cells into building materials, such as glazing and shading devices. BIPV can generate electricity while providing shading, reducing the need for separate solar panels. BIPV systems are expected to become more common in the future, as they offer both energy generation and shading benefits. The challenge is to achieve high efficiency with aesthetic integration.
Many thanks to our sponsor Focus 360 Energy who helped us prepare this research report.
9. Conclusion
Climate plays a critical role in the design and implementation of effective solar shading strategies. Understanding the specific climatic conditions of a region is essential for selecting appropriate shading materials, designs, and control strategies. This report has provided a comprehensive overview of solar shading strategies across diverse climatic zones, highlighting the challenges and opportunities presented by each climate. The findings emphasize the significance of a climate-responsive design approach to solar shading, leading to improved occupant comfort, reduced energy consumption, and enhanced building sustainability. Future research should focus on the development of advanced shading technologies, such as smart materials and building-integrated photovoltaics, and the integration of these technologies into building design practices. In particular, more research should focus on the long-term performance of shading solutions with a particular focus on durability and maintenance requirements. A standardised rating system for solar shading solutions should be developed.
Many thanks to our sponsor Focus 360 Energy who helped us prepare this research report.
References
- ASHRAE Handbook – Fundamentals. (2017). American Society of Heating, Refrigerating and Air-Conditioning Engineers.
- Mills, G. (2011). Buying solar: a consumer guide to selecting and installing solar electric systems. New Society Publishers.
- Olgyay, V. (2015). Design with climate: Bioclimatic architectural regionalism. John Wiley & Sons.
- Pérez-Lombard, L., Ortiz, J., & Pout, C. (2008). A review on buildings energy consumption information. Energy and Buildings, 40(3), 394-398.
- Stein, B., Reynolds, J. S., & McGuinness, W. J. (2006). Mechanical and electrical equipment for buildings. John Wiley & Sons.
- Wigginton, M., & Harris, J. (2002). Intelligent skins. Architectural Press.
- Wong, N. H., Li, S., & Yu, R. (2008). Performance evaluation of rooftop greenery systems in Singapore. Energy and Buildings, 40(12), 2194-2204.
- https://www.energy.gov/eere/buildings/solar-shading
- https://www.wbdg.org/design-objectives/sustainable/optimize-energy-performance
- https://www.ies.org/
The report highlights material durability in harsh climates. Could you elaborate on specific testing methodologies used to assess the long-term performance and weathering of different shading materials under varying climate conditions?
That’s a great question! We used a combination of accelerated weathering tests (like UV exposure and humidity cycling) and real-world field studies in various harsh climate locations. We monitored changes in colour, strength, and structural integrity over extended periods to simulate long-term performance. These testing methodologies are further detailed in the appendix of the full report!
Editor: FocusNews.Uk
Thank you to our Sponsor Focus 360 Energy
Fascinating report! Given the increasing frequency of extreme weather events, how do these shading strategies hold up against things like hailstorms or freak snowfalls, especially concerning long-term structural integrity and maintenance costs?
Thanks for the great question! The impact of extreme weather is definitely a key consideration. We found that material selection and design detailing are crucial for resilience. Exploring innovative materials that can withstand these events while minimizing maintenance is a key area for future research and development. What materials do you think hold the most promise?
Editor: FocusNews.Uk
Thank you to our Sponsor Focus 360 Energy
Interesting report. The discussion of dynamic shading systems and advanced control is particularly relevant. How do you see the integration of AI and machine learning impacting the responsiveness and adaptability of these shading systems in real-world applications, especially considering the complexities of varying occupancy patterns?
Thanks for your insightful question! AI and machine learning hold immense potential. Imagine shading systems learning building occupants’ preferences and automatically adjusting based on predicted usage patterns, leading to optimized energy savings and individual comfort. This adaptive approach could revolutionize building management!
Editor: FocusNews.Uk
Thank you to our Sponsor Focus 360 Energy