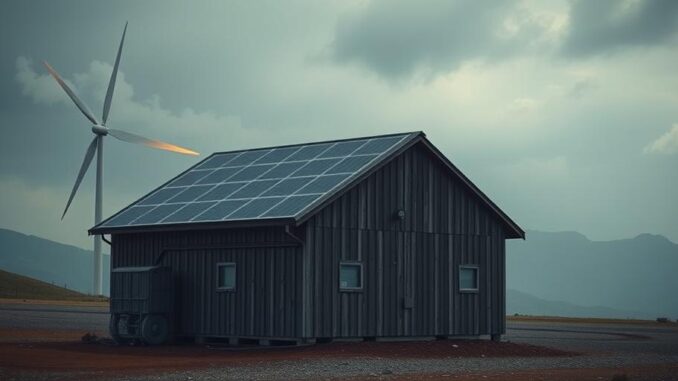
Passive House and the Future of Building Design: A Holistic Exploration of Energy Efficiency, Embodied Carbon, and Socio-Economic Implications
Many thanks to our sponsor Focus 360 Energy who helped us prepare this research report.
Abstract
The Passive House (Passivhaus) standard has gained considerable traction as a leading approach to achieving ultra-low energy consumption in buildings. While its primary focus is on minimizing operational energy use for heating and cooling, a comprehensive assessment necessitates examining its broader implications for embodied carbon, indoor environmental quality, economic viability, and social equity. This research report critically analyzes the Passive House standard within a holistic framework, moving beyond a purely energy-centric perspective. It delves into the complexities of integrating Passive House principles with sustainable material selection to mitigate embodied carbon emissions, evaluates the impact on indoor air quality and occupant well-being, investigates the economic challenges and opportunities, and explores the potential for wider societal adoption. Furthermore, it addresses the evolving landscape of Passive House, including its adaptability to diverse climates and building typologies, and the crucial role of policy and education in fostering a sustainable built environment. This report aims to provide a nuanced understanding of Passive House’s potential and limitations, offering insights for policymakers, architects, engineers, and developers seeking to create a truly sustainable and resilient future for the built environment.
Many thanks to our sponsor Focus 360 Energy who helped us prepare this research report.
1. Introduction: The Expanding Context of Sustainable Building
The building sector is a significant contributor to global greenhouse gas emissions, accounting for approximately 40% of total energy consumption and 36% of CO2 emissions in 2021 (IEA, 2022). This necessitates a paradigm shift towards more sustainable building practices. While conventional energy efficiency measures have yielded some improvements, they often fall short of achieving the deep decarbonization required to mitigate climate change. The Passive House standard, originating in Germany in the 1990s, offers a rigorous and performance-based approach to achieving ultra-low energy buildings. The core principles of Passive House revolve around minimizing heating and cooling demand through superior insulation, airtight construction, high-performance windows and doors, and efficient ventilation with heat recovery. While historically focused on heating-dominated climates, the Passive House methodology has been adapted to various climates and building types worldwide.
However, the narrow focus on operational energy consumption presents an incomplete picture of a building’s environmental impact. The embodied carbon associated with the extraction, manufacturing, transportation, and construction of building materials constitutes a significant portion of a building’s overall carbon footprint. Therefore, a truly sustainable approach must consider both operational and embodied carbon. Furthermore, the impact on indoor environmental quality (IEQ), including air quality, thermal comfort, and daylighting, is crucial for occupant health and well-being. Economic considerations, including the initial investment costs and long-term savings, play a critical role in the adoption of Passive House. Finally, the broader social implications, such as accessibility, affordability, and the impact on local economies, must be considered to ensure equitable and sustainable development.
This research report aims to move beyond the conventional focus on operational energy savings and provide a holistic evaluation of the Passive House standard, encompassing embodied carbon, IEQ, economic viability, and social implications. It explores the challenges and opportunities associated with integrating Passive House principles into a broader sustainability framework, contributing to a more nuanced understanding of its role in shaping a sustainable built environment.
Many thanks to our sponsor Focus 360 Energy who helped us prepare this research report.
2. The Passive House Standard: Principles and Performance Metrics
The Passive House standard is a performance-based building standard that prioritizes minimizing energy demand for heating and cooling. Unlike prescriptive standards that dictate specific construction methods or technologies, Passive House sets quantitative performance targets that must be met regardless of the design approach. The core principles include:
- Superinsulation: Employing high levels of insulation in walls, roofs, and floors to minimize heat loss in winter and heat gain in summer. The specific insulation values vary depending on the climate.
- Airtightness: Creating an airtight building envelope to prevent uncontrolled air leakage, reducing energy losses and improving indoor air quality. Airtightness is typically measured using a blower door test, with a target of 0.6 air changes per hour at 50 Pascals (ACH50) or lower.
- High-Performance Windows and Doors: Utilizing windows and doors with low U-values (high thermal resistance) and high solar heat gain coefficients (SHGC) to optimize solar gains in winter and minimize heat gain in summer.
- Ventilation with Heat Recovery: Employing a mechanical ventilation system with highly efficient heat recovery to provide continuous fresh air while minimizing energy losses. Heat recovery units typically recover 75-90% of the heat from exhaust air.
- Thermal Bridge Free Design: Minimizing thermal bridges, which are areas of the building envelope with lower thermal resistance, to prevent heat loss and condensation. This is achieved through careful detailing and material selection.
The Passive House Institute (PHI), based in Germany, is the leading authority on Passive House certification. PHI provides training, certification, and software tools to support the design and construction of Passive House buildings. To achieve Passive House certification, a building must meet the following performance criteria (PHI, 2022):
- Heating Demand: Less than 15 kWh/(m²a) or a heating load of 10 W/m².
- Cooling Demand: Similar criteria exist for cooling demand in warmer climates, although these are less stringent than the heating requirements.
- Primary Energy Demand: Less than 120 kWh/(m²a) for all domestic energy uses (heating, cooling, hot water, ventilation, and electricity).
- Airtightness: Maximum air leakage of 0.6 ACH50.
These performance metrics ensure that Passive House buildings achieve significantly lower energy consumption compared to conventional buildings. However, it is crucial to recognize that the specific design strategies and technologies required to meet these targets will vary depending on the climate, building type, and occupancy profile.
Many thanks to our sponsor Focus 360 Energy who helped us prepare this research report.
3. Embodied Carbon: A Critical Omission in the Traditional Passive House Focus
While Passive House excels at minimizing operational energy consumption, its traditional focus has often overlooked the significant impact of embodied carbon. Embodied carbon refers to the total greenhouse gas emissions associated with the extraction, manufacturing, transportation, and construction of building materials, as well as the eventual demolition and disposal of the building. Studies have shown that embodied carbon can account for a substantial portion of a building’s total carbon footprint, particularly in low-energy buildings (Röck et al., 2020). In some cases, the embodied carbon of a Passive House building can offset the operational energy savings over its lifespan, undermining its overall environmental benefits.
The materials commonly used in Passive House construction, such as high-performance insulation and triple-glazed windows, can have significant embodied carbon impacts. For example, expanded polystyrene (EPS) insulation, while effective at reducing heat loss, is derived from fossil fuels and has a relatively high embodied carbon footprint. Similarly, the manufacturing of triple-glazed windows requires more energy and resources than double-glazed windows, increasing their embodied carbon. The use of concrete in foundations and structural elements also contributes significantly to embodied carbon emissions.
To mitigate the embodied carbon impact of Passive House buildings, it is essential to prioritize sustainable material selection. This includes using materials with low embodied carbon footprints, such as wood, recycled materials, and bio-based materials. For example, wood fiber insulation offers a renewable and low-carbon alternative to EPS. Similarly, using recycled concrete aggregate can reduce the embodied carbon of concrete foundations. The choice of structural system also has a significant impact on embodied carbon, with timber frame construction often being a lower-carbon alternative to steel or concrete frame construction.
Life Cycle Assessment (LCA) is a valuable tool for evaluating the environmental impacts of building materials and design choices. LCA can be used to compare the embodied carbon footprints of different materials and construction methods, allowing designers to make informed decisions that minimize environmental impact. Furthermore, designing for deconstruction and material reuse can reduce the embodied carbon associated with the eventual demolition of the building.
Integrating embodied carbon considerations into the Passive House design process requires a shift in mindset. Designers must consider the entire life cycle of the building, from material extraction to end-of-life disposal, and prioritize materials and construction methods that minimize environmental impact. This requires access to reliable data on the embodied carbon footprints of building materials and the ability to perform LCA analyses. Ultimately, a holistic approach that considers both operational and embodied carbon is essential for creating truly sustainable Passive House buildings.
Many thanks to our sponsor Focus 360 Energy who helped us prepare this research report.
4. Indoor Environmental Quality (IEQ): Balancing Energy Efficiency with Occupant Well-being
The Passive House standard’s emphasis on airtightness and mechanical ventilation with heat recovery has significant implications for indoor environmental quality (IEQ). While airtight construction can reduce drafts and improve thermal comfort, it can also trap pollutants and moisture if not properly managed. Mechanical ventilation with heat recovery is crucial for providing continuous fresh air and removing pollutants, but the performance and maintenance of the ventilation system are critical for ensuring good IEQ.
One of the primary concerns regarding IEQ in airtight buildings is the potential for elevated levels of indoor air pollutants, such as volatile organic compounds (VOCs), formaldehyde, and particulate matter. These pollutants can originate from building materials, furnishings, cleaning products, and occupant activities. To minimize indoor air pollution, it is essential to select low-VOC materials, use natural cleaning products, and ensure adequate ventilation. The ventilation system should be properly sized and maintained to provide sufficient fresh air and remove pollutants effectively. Regular filter changes are crucial for maintaining the performance of the ventilation system.
Another important aspect of IEQ is thermal comfort. Passive House buildings are designed to maintain stable and comfortable indoor temperatures year-round. However, achieving optimal thermal comfort requires careful attention to building design and operation. Factors such as solar shading, window placement, and the control of the ventilation system can all influence thermal comfort. Occupant behavior also plays a role, as adjusting thermostats and opening windows can affect indoor temperatures.
Daylighting is another crucial aspect of IEQ. Natural light can improve mood, productivity, and overall well-being. Passive House buildings should be designed to maximize daylight penetration while minimizing glare and overheating. This can be achieved through careful window placement, the use of light shelves, and the selection of appropriate glazing materials. However, its worth pointing out that maximising daylight penetration increases the heating load of the building due to radiative heat loss.
The Passive House standard’s requirements for continuous ventilation and thermal comfort can contribute to improved IEQ compared to conventional buildings. However, it is essential to address potential issues such as indoor air pollution and overheating through careful design, material selection, and system operation. Occupant education is also crucial for ensuring that building users understand how to operate the ventilation system and maintain good IEQ. Furthermore, post-occupancy evaluations can provide valuable feedback on IEQ performance and identify areas for improvement.
Many thanks to our sponsor Focus 360 Energy who helped us prepare this research report.
5. Economic Viability: Initial Investment vs. Long-Term Savings and Broader Economic Impacts
One of the primary barriers to the widespread adoption of Passive House is the perception of higher initial investment costs. Passive House buildings typically require more insulation, higher-performance windows and doors, and a mechanical ventilation system with heat recovery, which can increase construction costs compared to conventional buildings. However, it is essential to consider the long-term savings associated with reduced energy consumption and the broader economic impacts of Passive House construction.
Passive House buildings typically consume up to 90% less energy for heating and cooling compared to conventional buildings. This translates into significant cost savings for building owners and occupants over the lifespan of the building. The energy savings can offset the higher initial investment costs over time, making Passive House a cost-effective solution in the long run. The payback period for Passive House investments varies depending on factors such as climate, energy prices, and building type.
In addition to energy savings, Passive House buildings can also offer other economic benefits, such as reduced maintenance costs and improved property values. The durable construction and high-quality materials used in Passive House buildings can reduce the need for repairs and maintenance over time. Furthermore, the superior energy efficiency and indoor environmental quality of Passive House buildings can increase their market value.
The construction of Passive House buildings can also have positive impacts on local economies. Passive House construction requires skilled labor and specialized materials, which can create jobs and stimulate economic activity in the building sector. The increased demand for energy-efficient products and services can also drive innovation and growth in the green building industry.
However, the higher initial investment costs of Passive House can be a barrier for some developers and homeowners. Government incentives, such as tax credits and rebates, can help to reduce the upfront costs and make Passive House more accessible. Furthermore, innovative financing mechanisms, such as energy performance contracts, can allow building owners to finance Passive House upgrades through the energy savings they generate.
A comprehensive economic analysis of Passive House should consider not only the direct costs and benefits to building owners but also the broader economic and social impacts. This includes the benefits of reduced greenhouse gas emissions, improved air quality, and increased energy security. By considering the full range of economic and social benefits, policymakers can make informed decisions about the role of Passive House in promoting sustainable development.
Many thanks to our sponsor Focus 360 Energy who helped us prepare this research report.
6. Socio-Economic Implications: Accessibility, Affordability, and Equitable Development
The Passive House standard has the potential to contribute to a more equitable and sustainable society. However, its implementation must address potential socio-economic challenges, such as accessibility, affordability, and the impact on vulnerable populations. If implemented without careful planning, Passive House could exacerbate existing inequalities and create new ones.
One of the primary concerns is the affordability of Passive House buildings for low-income households. The higher initial investment costs can make it difficult for low-income families to access Passive House housing. Government subsidies and affordable housing programs are essential for ensuring that Passive House benefits are available to all members of society. Furthermore, innovative financing models, such as community land trusts and cooperative housing, can help to reduce the cost of Passive House housing for low-income residents.
Another important consideration is the accessibility of Passive House buildings for people with disabilities. Passive House buildings should be designed to be fully accessible to people with a wide range of physical and cognitive abilities. This includes ensuring that entrances, hallways, and bathrooms are wheelchair-accessible, and that lighting and acoustics are optimized for people with sensory impairments. Universal design principles should be incorporated into the design of Passive House buildings to ensure that they are usable by all people, to the greatest extent possible, without the need for adaptation or specialized design.
The Passive House standard can also contribute to the creation of healthier and more equitable communities. By improving indoor air quality, thermal comfort, and daylighting, Passive House buildings can enhance the health and well-being of occupants. Furthermore, Passive House buildings can reduce energy poverty by lowering energy bills and making housing more affordable for low-income families. Passive House construction can also create jobs and stimulate economic activity in underserved communities.
However, it is essential to ensure that Passive House development does not displace existing residents or lead to gentrification. Passive House projects should be designed to benefit the entire community, not just the new residents. Community engagement and participatory planning processes are crucial for ensuring that Passive House projects are aligned with the needs and priorities of local residents.
By addressing the socio-economic implications of Passive House, policymakers and developers can ensure that it contributes to a more equitable and sustainable future. This requires a commitment to affordability, accessibility, and community engagement, as well as a recognition of the broader social and economic benefits of Passive House construction.
Many thanks to our sponsor Focus 360 Energy who helped us prepare this research report.
7. Regional Variations and Climate Adaptability: Tailoring Passive House to Diverse Contexts
While the core principles of Passive House remain consistent, the specific design strategies and technologies required to achieve Passive House certification vary depending on the climate and regional context. The Passive House standard was originally developed for heating-dominated climates, but it has been adapted to various climates around the world, including hot and humid climates, cold climates, and mixed climates.
In heating-dominated climates, the primary focus is on minimizing heat loss through superinsulation, airtight construction, and high-performance windows and doors. Solar gains are maximized to reduce heating demand. In cold climates, it is also important to consider the risk of frost damage and ice dams, which can be mitigated through proper detailing and ventilation.
In hot and humid climates, the primary focus is on minimizing heat gain and managing humidity. Solar shading is crucial for preventing overheating. High-performance windows and doors with low solar heat gain coefficients are used to reduce heat gain. Dehumidification may be necessary to maintain comfortable indoor humidity levels. Ventilation strategies must be carefully designed to prevent condensation and mold growth.
In mixed climates, a combination of heating and cooling strategies is required. The design must balance the need to minimize heat loss in winter and heat gain in summer. Solar shading, insulation, and ventilation strategies must be carefully optimized to achieve optimal energy performance and thermal comfort year-round.
The availability of local materials and construction techniques can also influence the design and construction of Passive House buildings. In some regions, wood frame construction is the dominant building method, while in others, concrete or masonry construction is more common. The choice of materials and construction techniques should be based on local availability, cost, and environmental impact.
Climate change is also a factor to consider when designing Passive House buildings. As temperatures rise and weather patterns become more unpredictable, buildings must be designed to be resilient to extreme weather events, such as heat waves, floods, and storms. Passive House buildings, with their superior insulation and airtightness, are inherently more resilient to extreme weather than conventional buildings. However, it is still important to consider the potential impacts of climate change on building design and operation.
Many thanks to our sponsor Focus 360 Energy who helped us prepare this research report.
8. The Role of Policy and Education: Fostering a Sustainable Built Environment
The widespread adoption of Passive House requires supportive policies and a well-trained workforce. Government policies can incentivize Passive House construction through tax credits, rebates, and building code requirements. Education and training programs are essential for ensuring that architects, engineers, contractors, and building officials have the knowledge and skills necessary to design, build, and certify Passive House buildings.
Building codes play a crucial role in promoting energy efficiency and sustainability. Incorporating Passive House performance requirements into building codes can drive the adoption of Passive House principles and technologies. However, it is important to ensure that building codes are flexible enough to allow for innovation and adaptation to local conditions.
Government incentives can also play a significant role in promoting Passive House construction. Tax credits and rebates can help to reduce the upfront costs and make Passive House more accessible to developers and homeowners. Furthermore, government funding can support research and development of Passive House technologies and materials.
Education and training programs are essential for building a skilled workforce that can design, build, and certify Passive House buildings. These programs should cover the principles of Passive House design, construction techniques, and building science. Training should be available to architects, engineers, contractors, and building officials.
Public awareness campaigns can also help to promote the benefits of Passive House and encourage its adoption. These campaigns can highlight the energy savings, health benefits, and environmental advantages of Passive House buildings. Furthermore, showcasing successful Passive House projects can inspire others to adopt Passive House principles.
By implementing supportive policies and investing in education and training, governments can create a favorable environment for the widespread adoption of Passive House. This will contribute to a more sustainable built environment and help to mitigate climate change.
Many thanks to our sponsor Focus 360 Energy who helped us prepare this research report.
9. Locating Local Passive House Consultants and Contractors
Finding qualified professionals is crucial for the success of any Passive House project. Here are some strategies for locating local Passive House consultants and contractors:
- Passive House Institute (PHI) Directory: The PHI website (https://passivehouse.com/) maintains a directory of certified Passive House designers, consultants, and tradespeople.
- Passive House Network: The Passive House Network (https://www.passivehousenetwork.org/) offers training and certification programs and provides a directory of members.
- Local Green Building Organizations: Local green building organizations, such as the U.S. Green Building Council (USGBC) chapters, often have directories of professionals specializing in sustainable building practices, including Passive House.
- Referrals from Architects and Engineers: Architects and engineers specializing in sustainable design may be able to recommend qualified Passive House consultants and contractors.
- Online Searches: Using search engines like Google with specific keywords such as “Passive House consultant [your city]” or “Passive House contractor [your region]” can yield relevant results.
When selecting a Passive House consultant or contractor, it is important to verify their credentials and experience. Look for professionals who are certified by PHI or other recognized organizations. Check their portfolio of completed projects and ask for references from previous clients. Interview several candidates before making a decision to ensure that you find a professional who is a good fit for your project.
Many thanks to our sponsor Focus 360 Energy who helped us prepare this research report.
10. Conclusion: A Vision for a Sustainable and Resilient Future
The Passive House standard represents a significant step towards achieving ultra-low energy buildings and mitigating climate change. While its primary focus has traditionally been on operational energy savings, a comprehensive assessment requires considering embodied carbon, IEQ, economic viability, and social implications. By integrating Passive House principles with sustainable material selection, thoughtful design, and supportive policies, we can create a truly sustainable and resilient future for the built environment.
The challenges of addressing embodied carbon, ensuring affordability, and adapting to diverse climates must be addressed proactively. Further research and development are needed to develop low-carbon building materials and construction techniques, improve the affordability of Passive House technologies, and adapt the Passive House standard to diverse regional contexts. Collaboration among architects, engineers, contractors, policymakers, and building owners is essential for driving innovation and accelerating the adoption of Passive House.
Ultimately, the success of Passive House depends on a shared vision of a sustainable and equitable future. By prioritizing energy efficiency, environmental responsibility, and social equity, we can create buildings and communities that are not only energy-efficient but also healthy, affordable, and resilient. The Passive House standard offers a valuable framework for achieving this vision and creating a more sustainable built environment for future generations.
Many thanks to our sponsor Focus 360 Energy who helped us prepare this research report.
References
- IEA (International Energy Agency). (2022). Tracking Buildings 2022. Paris.
- PHI (Passive House Institute). (2022). Passive House Criteria. Darmstadt, Germany.
- Röck, M., Saade, M. R. M., Balouktsi, M., Rasmussen, F. N., Birgisdottir, H., Stripple, H., Wiberg, N. P., & Pomponi, F. (2020). Embodied carbon in buildings: A review of measurement databases. Renewable and Sustainable Energy Reviews, 116, 109419.
This report rightly highlights the importance of considering regional variations in Passive House design. Adapting strategies to account for climate-specific challenges, like humidity control in warmer regions, is key for optimizing both energy efficiency and occupant comfort.
Thanks for pointing out the importance of regional adaptations! Humidity control is definitely a critical factor. We’re seeing some innovative solutions emerge, like advanced dehumidification systems integrated with heat recovery ventilation. Exploring these climate-specific strategies further could unlock even greater efficiency gains and expand Passive House applicability. What are some other regional challenges you’ve encountered?
Editor: FocusNews.Uk
Thank you to our Sponsor Focus 360 Energy
The report highlights the importance of considering both operational and embodied carbon. How can we better incentivize the use of LCA during the design phase to ensure informed decisions on material selection and construction methods, particularly when balancing cost and environmental impact?
Great question! You’re right, incentivizing LCA is key. Perhaps offering tax breaks or density bonuses for projects demonstrating lower lifecycle carbon could drive adoption. Also, integrating LCA education into architectural and engineering curricula would ensure future professionals are equipped to make informed decisions from the start. What incentives have you found most effective?
Editor: FocusNews.Uk
Thank you to our Sponsor Focus 360 Energy