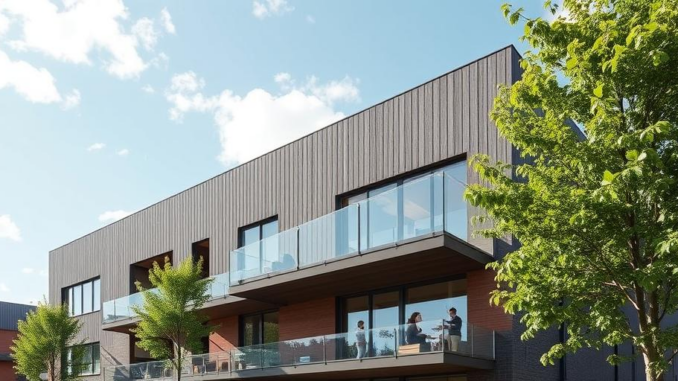
The Evolving Building Envelope: Integrating Performance, Resilience, and Sustainability in a Changing Climate
Abstract
The building envelope serves as the primary interface between the interior environment and the external climate, profoundly impacting energy consumption, indoor environmental quality (IEQ), and structural integrity. This research report provides a comprehensive overview of the evolving landscape of building envelope design, moving beyond a singular focus on energy efficiency to encompass resilience against climate change impacts and the imperative of sustainable material choices. We examine advanced insulation materials and techniques, explore the role of adaptive building skins in modulating environmental conditions, and delve into the complex interplay between material selection, embodied carbon, and long-term performance. Furthermore, the report addresses the integration of smart technologies for real-time monitoring and control of envelope performance and discusses the economic and regulatory drivers shaping the future of building envelope design. Through a critical analysis of current trends and emerging technologies, this report aims to provide architects, engineers, and policymakers with insights necessary to design building envelopes that are not only energy-efficient but also resilient, sustainable, and adaptable to the challenges of a rapidly changing world.
1. Introduction
The building envelope, comprising the walls, roof, fenestration, and foundation, is a critical component of any structure, acting as a selective filter that mediates the flow of energy, air, moisture, and light between the interior and exterior environments. Its design and construction significantly influence a building’s energy performance, indoor environmental quality (IEQ), durability, and resilience. Historically, the focus of building envelope design has largely been on minimizing energy consumption for heating and cooling. However, in the face of escalating climate change impacts, stricter energy codes, and growing awareness of the environmental footprint of the built environment, a more holistic approach is required. This approach must integrate energy efficiency with resilience, sustainability, and adaptability.
This research report aims to provide a comprehensive overview of the current state-of-the-art in building envelope design, construction, and performance assessment. We will explore advancements in materials science, construction techniques, and digital technologies that are transforming the way building envelopes are conceived and realized. Furthermore, we will examine the trade-offs between competing performance objectives, such as energy efficiency, thermal comfort, structural integrity, and environmental impact, highlighting the need for integrated design strategies that optimize building envelope performance across multiple dimensions. The report will also address the economic and regulatory drivers shaping the future of building envelope design, considering the role of government incentives, building codes, and market demand in promoting the adoption of innovative and sustainable envelope solutions.
2. Advanced Insulation Materials and Techniques
Insulation is a cornerstone of high-performance building envelopes, playing a crucial role in reducing heat transfer through conduction, convection, and radiation. Traditional insulation materials, such as fiberglass, mineral wool, and polystyrene, have been widely used for decades due to their relatively low cost and ease of installation. However, these materials often have limitations in terms of thermal performance, moisture resistance, and environmental impact. As a result, there is a growing demand for advanced insulation materials that offer improved thermal properties, enhanced durability, and reduced environmental footprint.
2.1 Emerging Insulation Materials:
-
Aerogels: Aerogels are highly porous, solid materials derived from a gel in which the liquid component has been replaced with a gas. They exhibit extremely low thermal conductivity, making them one of the most effective insulation materials available. While traditionally expensive, recent advances in manufacturing techniques are making aerogels more cost-competitive for building envelope applications. Aerogel-based insulation can be incorporated into blankets, panels, and coatings, offering versatile solutions for both new construction and retrofit projects.
-
Vacuum Insulation Panels (VIPs): VIPs consist of a rigid core material encased in an airtight envelope, with the air evacuated to create a near-vacuum environment. This drastically reduces heat transfer through conduction and convection, resulting in exceptionally high thermal resistance. VIPs are particularly well-suited for applications where space is limited, such as exterior wall retrofits or balcony insulation. However, their fragility and susceptibility to damage during installation remain challenges.
-
Phase Change Materials (PCMs): PCMs are substances that absorb and release thermal energy during a phase transition (e.g., solid to liquid). When integrated into building envelope components, PCMs can help to stabilize indoor temperatures, reduce peak heating and cooling loads, and improve thermal comfort. PCMs can be incorporated into wallboards, concrete, or insulation materials, offering a passive means of thermal energy storage.
-
Bio-Based Insulation Materials: Bio-based insulation materials, such as cellulose, hempcrete, sheep’s wool, and straw bales, are derived from renewable resources and offer a lower environmental impact compared to conventional insulation materials. These materials often have good thermal performance, moisture buffering capacity, and acoustic properties. However, their availability, cost, and regulatory acceptance may vary depending on the region.
2.2 Advanced Insulation Techniques:
-
Continuous Insulation (CI): Continuous insulation involves installing a layer of insulation that is continuous across all structural members, eliminating thermal bridging and improving overall thermal performance. CI can be achieved using rigid insulation boards, spray foam, or other insulation materials. It is particularly effective in reducing energy losses through walls and roofs.
-
Structural Insulated Panels (SIPs): SIPs are prefabricated building panels consisting of an insulating core sandwiched between two structural facings, typically oriented strand board (OSB). SIPs offer excellent thermal performance, structural strength, and air tightness. They can be used for walls, roofs, and floors, and can significantly reduce construction time and labor costs.
-
Spray Foam Insulation: Spray foam insulation is a versatile material that can be used to fill cavities, seal air leaks, and provide continuous insulation. There are two main types of spray foam: open-cell and closed-cell. Closed-cell spray foam offers higher R-value and better moisture resistance but is more expensive than open-cell foam.
The selection of appropriate insulation materials and techniques should be based on a comprehensive assessment of factors such as climate, building type, occupancy, budget, and environmental goals. In addition, proper installation is crucial to ensure that insulation performs as intended and achieves its full potential.
3. Airtight Construction and Air Leakage Control
Airtight construction is essential for achieving high-performance building envelopes and minimizing energy losses due to air leakage. Air leakage can account for a significant portion of a building’s heating and cooling loads, particularly in older buildings with poorly sealed envelopes. In addition to energy losses, air leakage can also contribute to moisture problems, indoor air quality issues, and reduced thermal comfort.
3.1 Sources of Air Leakage:
Air leakage can occur through a variety of pathways in the building envelope, including:
- Cracks and gaps around windows and doors
- Penetrations for pipes, wires, and ducts
- Gaps in sheathing and siding
- Unsealed joints between building components
- Chimneys and flues
3.2 Airtightness Testing:
Blower door testing is a common method for measuring the airtightness of a building. A blower door is a powerful fan that is installed in an exterior door opening to pressurize or depressurize the building. The airflow required to maintain a constant pressure difference between the interior and exterior is measured, providing an indication of the building’s air leakage rate. Airtightness is typically expressed in terms of air changes per hour at a pressure difference of 50 Pascals (ACH50).
3.3 Air Sealing Techniques:
Effective air sealing requires a systematic approach that addresses all potential sources of air leakage. Common air sealing techniques include:
- Caulking and sealing cracks and gaps around windows, doors, and penetrations
- Installing weather stripping around doors and windows
- Using spray foam to seal gaps and cavities
- Installing air barriers on the exterior or interior of the building envelope
- Sealing ductwork and pipe penetrations
3.4 Air Barrier Systems:
Air barriers are materials or assemblies that are designed to resist airflow through the building envelope. They can be installed on the exterior or interior of the building envelope, and they can be made from a variety of materials, including membranes, films, and coatings. Air barrier systems should be continuous, durable, and compatible with other building materials. Careful detailing and installation are crucial to ensure that the air barrier system performs effectively.
The pursuit of airtight construction should be balanced with the need for adequate ventilation. Mechanical ventilation systems are often required to provide fresh air and exhaust stale air, particularly in tightly sealed buildings. Heat recovery ventilators (HRVs) and energy recovery ventilators (ERVs) can be used to recover heat or energy from the exhaust air, reducing energy losses associated with ventilation.
4. Adaptive Building Skins: Responding to Dynamic Environmental Conditions
Adaptive building skins represent a paradigm shift in building envelope design, moving from static, passive systems to dynamic, responsive systems that can adapt to changing environmental conditions. These systems employ a variety of technologies and materials to modulate the flow of energy, air, light, and moisture through the building envelope, optimizing performance and enhancing occupant comfort.
4.1 Types of Adaptive Building Skins:
-
Dynamic Shading Devices: Dynamic shading devices, such as motorized shades, louvers, and fins, can be adjusted to control the amount of sunlight entering a building. This can reduce glare, improve thermal comfort, and reduce cooling loads. Dynamic shading devices can be controlled manually or automatically based on solar position, occupancy, and other factors.
-
Electrochromic Windows: Electrochromic windows are windows that can change their opacity in response to an electrical signal. This allows for dynamic control of solar heat gain and glare. Electrochromic windows can be used to reduce cooling loads and improve occupant comfort. However, their cost remains a barrier to widespread adoption.
-
Thermochromic Materials: Thermochromic materials are materials that change color in response to temperature changes. These materials can be used in coatings, glazings, and other building envelope components to provide passive control of solar heat gain. When the material reaches a certain temperature, it changes color to reflect more sunlight, reducing heat gain.
-
Kinetic Façades: Kinetic façades are building envelopes that incorporate moving parts, allowing them to dynamically adjust their shape, orientation, or properties. These façades can be used to control solar shading, ventilation, and aesthetics. Kinetic façades are often complex and expensive, but they can provide significant performance benefits and architectural interest.
-
Building-Integrated Photovoltaics (BIPV): BIPV systems integrate photovoltaic cells into building envelope components, such as roofing tiles, wall panels, and glazing. BIPV systems can generate electricity on-site, reducing reliance on grid power and lowering energy costs. In addition to generating electricity, BIPV systems can also provide shading and thermal insulation.
4.2 Control Strategies:
The effectiveness of adaptive building skins depends on the control strategies used to manage their operation. Control strategies can be based on a variety of factors, including:
- Solar position: Tracking the sun’s movement and adjusting shading devices to optimize solar control.
- Occupancy: Adjusting lighting and ventilation based on occupancy patterns.
- Weather conditions: Responding to changes in temperature, humidity, and precipitation.
- Energy prices: Adjusting energy consumption based on real-time energy prices.
Advanced control systems can use sensors, actuators, and algorithms to optimize the performance of adaptive building skins. Machine learning techniques can be used to predict building energy consumption and adjust control strategies accordingly.
5. Material Selection, Embodied Carbon, and Life Cycle Assessment
Traditionally, building envelope material selection has been primarily driven by cost, structural performance, and aesthetic considerations. However, with growing awareness of the environmental impact of the built environment, there is an increasing emphasis on sustainable material choices that minimize embodied carbon and promote life cycle thinking.
5.1 Embodied Carbon:
Embodied carbon refers to the greenhouse gas emissions associated with the extraction, manufacturing, transportation, and installation of building materials. It represents a significant portion of a building’s overall carbon footprint, particularly in energy-efficient buildings where operational energy use is minimized. Reducing embodied carbon requires careful consideration of material selection and sourcing.
5.2 Life Cycle Assessment (LCA):
Life Cycle Assessment (LCA) is a methodology for evaluating the environmental impacts of a product or service throughout its entire life cycle, from raw material extraction to end-of-life disposal. LCA can be used to compare the environmental performance of different building materials and envelope designs, taking into account factors such as embodied carbon, energy consumption, water usage, and waste generation.
5.3 Sustainable Material Choices:
-
Low-Carbon Concrete: Concrete is a widely used building material, but its production is a significant source of greenhouse gas emissions. Low-carbon concrete can be achieved by using supplementary cementitious materials (SCMs) such as fly ash, slag, or silica fume to replace a portion of the Portland cement. Other strategies include using recycled aggregates and optimizing concrete mix designs.
-
Mass Timber: Mass timber products, such as cross-laminated timber (CLT), glued laminated timber (glulam), and laminated veneer lumber (LVL), offer a sustainable alternative to concrete and steel for structural applications. Mass timber is made from renewable wood resources and can sequester carbon dioxide from the atmosphere. However, responsible forest management practices are essential to ensure the sustainability of mass timber.
-
Recycled and Reclaimed Materials: Using recycled and reclaimed materials in building envelope construction can reduce embodied carbon and minimize waste. Examples include recycled steel, reclaimed wood, and recycled plastic insulation.
-
Bio-Based Materials: As discussed earlier, bio-based insulation materials, such as cellulose, hempcrete, and straw bales, offer a lower environmental impact compared to conventional materials. These materials are derived from renewable resources and can sequester carbon dioxide from the atmosphere.
The selection of sustainable building envelope materials should be based on a comprehensive LCA that considers the environmental impacts of the entire life cycle. It is also important to consider the durability, performance, and cost of the materials.
6. Smart Technologies for Envelope Monitoring and Control
The integration of smart technologies into building envelopes is enabling real-time monitoring and control of envelope performance, leading to improved energy efficiency, enhanced indoor environmental quality, and increased occupant comfort. Sensors, actuators, and control systems can be used to optimize the operation of adaptive building skins, detect air leaks, and monitor moisture levels.
6.1 Sensor Technologies:
-
Temperature Sensors: Temperature sensors can be used to monitor the temperature of building envelope surfaces, air cavities, and indoor spaces. This information can be used to optimize heating and cooling systems and to detect thermal bridges.
-
Humidity Sensors: Humidity sensors can be used to monitor the moisture content of building envelope materials and indoor air. This information can be used to detect moisture problems, such as condensation and mold growth.
-
Airflow Sensors: Airflow sensors can be used to measure air leakage rates and to monitor the performance of ventilation systems.
-
Light Sensors: Light sensors can be used to measure the amount of sunlight entering a building. This information can be used to optimize lighting control systems and to adjust shading devices.
6.2 Control Systems:
Control systems can be used to automatically adjust the operation of building envelope components based on sensor data, occupancy patterns, weather conditions, and other factors. Advanced control systems can use machine learning techniques to predict building energy consumption and optimize control strategies.
6.3 Building Management Systems (BMS):
Building Management Systems (BMS) are integrated control systems that can monitor and control a wide range of building systems, including HVAC, lighting, and security. BMS can be used to optimize the performance of the building envelope and to provide real-time feedback to building operators.
The use of smart technologies in building envelopes is still in its early stages, but it has the potential to revolutionize the way buildings are designed and operated. As sensor technologies become more affordable and control systems become more sophisticated, we can expect to see a wider adoption of smart envelope solutions.
7. Economic and Regulatory Drivers
The adoption of high-performance building envelopes is influenced by a variety of economic and regulatory drivers, including government incentives, building codes, and market demand.
7.1 Government Incentives:
Government incentives, such as tax credits, rebates, and grants, can help to offset the upfront costs of high-performance building envelopes. These incentives can encourage building owners and developers to invest in energy-efficient and sustainable building solutions.
7.2 Building Codes:
Building codes, such as the International Energy Conservation Code (IECC) and ASHRAE Standard 90.1, set minimum energy efficiency standards for building envelopes. These codes are becoming increasingly stringent, driving the adoption of advanced insulation materials, airtight construction techniques, and high-performance windows.
7.3 Market Demand:
Market demand for energy-efficient and sustainable buildings is also driving the adoption of high-performance building envelopes. Building owners and occupants are increasingly aware of the benefits of green buildings, including lower energy costs, improved indoor environmental quality, and enhanced market value.
The combination of government incentives, building codes, and market demand is creating a strong incentive for the adoption of high-performance building envelopes. As the costs of these technologies continue to decline and the benefits become more widely recognized, we can expect to see a wider adoption of sustainable and resilient building envelope solutions.
8. Future Trends and Research Directions
The field of building envelope design is constantly evolving, driven by advancements in materials science, construction techniques, and digital technologies. Some of the key future trends and research directions include:
-
Development of new materials with improved thermal performance, durability, and sustainability: Research is ongoing to develop new insulation materials, glazing technologies, and air barrier systems that offer improved performance and reduced environmental impact.
-
Integration of advanced control systems and artificial intelligence (AI) for real-time optimization of envelope performance: AI can be used to predict building energy consumption and adjust control strategies for adaptive building skins, ventilation systems, and other building systems.
-
Development of more robust and reliable methods for assessing building envelope performance: Improved testing methods and simulation tools are needed to accurately predict the performance of building envelopes under a wide range of conditions.
-
Development of standardized methods for quantifying the embodied carbon of building materials: Standardized LCA methodologies are needed to enable informed material selection decisions based on environmental impact.
-
Investigation of the long-term performance and durability of advanced building envelope systems: Long-term monitoring and testing are needed to ensure that advanced building envelope systems perform as intended over their entire life cycle.
-
Exploration of novel building envelope designs that integrate multiple functions, such as energy generation, water harvesting, and air purification: Multifunctional building envelopes can offer significant performance benefits and contribute to a more sustainable built environment.
9. Conclusion
The building envelope is a critical component of any structure, playing a crucial role in energy efficiency, indoor environmental quality, and structural integrity. This report has provided a comprehensive overview of the evolving landscape of building envelope design, moving beyond a singular focus on energy efficiency to encompass resilience against climate change impacts and the imperative of sustainable material choices. The integration of advanced insulation materials, airtight construction techniques, adaptive building skins, and smart technologies is enabling the creation of high-performance building envelopes that are not only energy-efficient but also resilient, sustainable, and adaptable to the challenges of a rapidly changing world. Continued research and development in this area are essential to further improve the performance and sustainability of building envelopes and to contribute to a more sustainable built environment.
References
- ASHRAE Standard 90.1-2019, Energy Standard for Buildings Except Low-Rise Residential Buildings.
- International Energy Conservation Code (IECC), 2021.
- Pacheco-Torgal, F., et al. (2014). Eco-efficient construction and building materials: life cycle assessment (LCA), Springer.
- Roodman, D. M., & Lenssen, N. (1995). A building revolution: How ecology and health concerns are transforming construction. Worldwatch Institute.
- Attia, S., et al. (2012). Overview of current research trends in adaptive building facades. Energy and Buildings, 48, 257-272.
- Holladay, D. (2019). Building science for building envelopes. John Wiley & Sons.
- GhaffarianHoseini, A., et al. (2016). A review on the significance of passive building design strategies for energy efficiency in buildings. Renewable and Sustainable Energy Reviews, 57, 1192-1217.
- Pomponi, F., & Moncaster, A. (2016). Embodied carbon for buildings: A review. Renewable and Sustainable Energy Reviews, 65, 483-494.
- Cabeza, L. F., et al. (2011). Phase change materials for energy efficiency and thermal comfort in buildings. Energy and Buildings, 43(3), 727-736.
- Jelle, B. P., & Hynd, A. W. (2011). Vacuum insulation panels for building applications: A review and future perspectives. Energy and Buildings, 43(10), 2577-2603.
- Buratti, C., & Moretti, L. (2012). Energy and environmental comparison of traditional insulation materials and innovative solutions for building applications. Energy and Buildings, 47, 245-253.
- Asdrubali, F., Baldinelli, G., & D’Alessandro, F. (2013). Life cycle assessment in the construction sector: Comparing innovative and traditional solutions. Energy and Buildings, 64, 392-399.
- Ding, W., et al. (2021). Adaptive building envelopes for energy efficiency and sustainability: A review. Building and Environment, 196, 107777.
Considering the growing emphasis on minimizing embodied carbon, how can we better leverage Life Cycle Assessments to inform material selection for building envelopes, especially in balancing regional availability with global sourcing impacts?