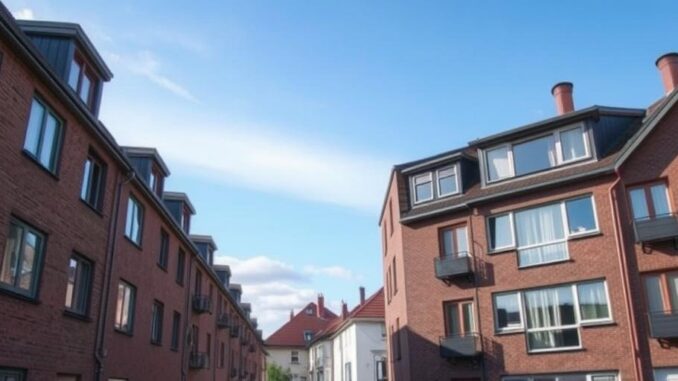
Abstract
Airtightness is increasingly recognized as a critical performance parameter for modern buildings, extending far beyond its traditional association with energy conservation. This research report provides a comprehensive review of airtightness in building envelopes, delving into the underlying physics of air leakage, advanced measurement techniques, and diverse strategies for enhancing airtightness. The scope extends beyond conventional practices to explore the complex interplay between airtightness, hygrothermal performance, indoor environmental quality (IEQ), and structural durability. Furthermore, the report examines the integration of airtightness strategies within a broader framework of sustainable building design, considering its influence on embodied carbon and life-cycle assessment. Finally, the report offers insights into the future of airtightness, including the development of smart building envelope technologies and the role of data-driven optimization in achieving high-performance building design.
Many thanks to our sponsor Focus 360 Energy who helped us prepare this research report.
1. Introduction
The building envelope serves as the primary barrier between the interior environment and the external climate. Its effectiveness in controlling heat transfer, moisture transport, and air leakage significantly impacts building energy consumption, occupant comfort, and long-term durability. While thermal insulation has long been emphasized as a key factor in reducing heat loss, the importance of airtightness is now widely acknowledged as an equally critical element in achieving high-performance building envelopes [1].
Air leakage through uncontrolled pathways in the building envelope can lead to substantial energy losses, drafts, and uncomfortable indoor environments. Furthermore, air leakage can facilitate moisture intrusion, potentially leading to mold growth, material degradation, and structural damage [2]. As building codes and energy efficiency standards become increasingly stringent, achieving high levels of airtightness is essential for compliance and for realizing the full potential of energy-saving measures.
This research report presents a comprehensive overview of airtightness in building envelopes, examining the underlying principles, measurement techniques, and strategies for improvement. The report goes beyond a simple discussion of energy savings, addressing the broader implications of airtightness for indoor air quality, building durability, and overall sustainability.
Many thanks to our sponsor Focus 360 Energy who helped us prepare this research report.
2. The Physics of Air Leakage
Air leakage is driven by pressure differences between the interior and exterior of a building. These pressure differences can be caused by a variety of factors, including:
- Stack effect: Warm air rises, creating a positive pressure at the top of the building and a negative pressure at the bottom.
- Wind pressure: Wind impinging on the windward side of a building creates positive pressure, while the leeward side experiences negative pressure.
- Mechanical systems: HVAC systems can create pressure imbalances within a building, driving air leakage.
- Buoyancy effects: Temperature differences between inside and outside of the building.
The rate of air leakage depends on the magnitude of the pressure difference and the size and number of air leakage pathways. Air leakage pathways can include cracks and gaps around windows and doors, penetrations for pipes and wiring, and imperfections in the building materials themselves [3].
The flow of air through these pathways can be described by the following equation:
Q = C * ΔP^n
where:
- Q is the air flow rate (e.g., m³/h or CFM)
- C is the air leakage coefficient, which depends on the size and shape of the leakage pathway
- ΔP is the pressure difference across the building envelope (e.g., Pa or inches of water)
- n is the flow exponent, which typically ranges from 0.5 to 1.0. A value of 0.5 indicates turbulent flow, while a value of 1.0 indicates laminar flow.
Understanding the physics of air leakage is crucial for identifying and addressing the root causes of air leakage in buildings. By minimizing pressure differences and sealing air leakage pathways, it is possible to significantly improve the airtightness of building envelopes.
Many thanks to our sponsor Focus 360 Energy who helped us prepare this research report.
3. Measuring Airtightness: Methods and Metrics
Several methods are available for measuring the airtightness of buildings. The most common method is the blower door test, which involves installing a powerful fan in an exterior door or window to create a pressure difference across the building envelope. The fan is used to pressurize or depressurize the building, and the airflow rate required to maintain a specific pressure difference is measured. This airflow rate is then used to calculate the air leakage rate of the building [4].
The results of blower door tests are typically expressed in terms of the following metrics:
- Air Changes per Hour at 50 Pascals (ACH50): This metric represents the number of times the air volume inside the building is replaced per hour when a pressure difference of 50 Pascals (Pa) is maintained between the inside and outside of the building. ACH50 is the most commonly used metric for assessing airtightness.
- Equivalent Leakage Area (ELA): This metric represents the total area of all the air leakage pathways in the building envelope. ELA is calculated based on the measured airflow rate and the assumed flow characteristics of the leakage pathways.
- Normalized Leakage Area (NLA): This metric is calculated by dividing the ELA by the building’s surface area. NLA provides a measure of airtightness that is independent of building size.
- Air Permeability (q50): Expressed as m3/(h.m2) at 50Pa. This metric represents the rate of air flow per unit area of the building envelope when subjected to a pressure difference of 50 Pascals.
Infrared thermography can be used in conjunction with blower door tests to identify specific air leakage pathways. Infrared cameras detect temperature differences on the surface of building materials, revealing areas where warm air is escaping or cold air is entering [5].
Other methods for measuring airtightness include tracer gas techniques and pressure box testing. Tracer gas techniques involve releasing a known concentration of tracer gas inside the building and measuring the rate at which the gas leaks out. Pressure box testing involves placing a small section of the building envelope inside a sealed chamber and measuring the airflow rate required to maintain a specific pressure difference across the section.
The selection of the appropriate measurement method depends on the specific application and the level of accuracy required. Blower door tests are generally considered the most reliable and practical method for measuring the airtightness of entire buildings, while tracer gas techniques and pressure box testing are more suitable for research purposes or for evaluating the airtightness of specific building components.
Many thanks to our sponsor Focus 360 Energy who helped us prepare this research report.
4. Strategies for Improving Airtightness
A variety of strategies can be employed to improve the airtightness of building envelopes. These strategies can be broadly categorized into the following areas:
- Sealing cracks and gaps: This involves identifying and sealing all cracks and gaps in the building envelope, including those around windows and doors, penetrations for pipes and wiring, and imperfections in the building materials themselves. Caulk, sealant, weather stripping, and expanding foam are commonly used to seal these pathways [6].
- Using air barriers: Air barriers are materials or assemblies that are specifically designed to resist air flow. Air barriers can be installed on the exterior or interior of the building envelope, and they should be continuous and sealed at all joints and penetrations [7]. Common air barrier materials include membranes, rigid boards, and spray-applied coatings.
- Minimizing penetrations: Reducing the number of penetrations in the building envelope can significantly improve airtightness. This can be achieved by carefully planning the layout of mechanical systems and electrical wiring, and by using prefabricated components with integrated air barriers.
- Detailing connections: The connections between different building components, such as walls and roofs, are often weak points in the air barrier system. Careful detailing and sealing of these connections is essential for achieving high levels of airtightness [8].
- Choosing materials: Certain building materials, such as concrete and closed-cell spray foam, are inherently more airtight than others. Selecting materials with good airtightness properties can simplify the process of achieving a tight building envelope.
- Consider prefabrication: Prefabricated building elements can be manufactured to a very high degree of airtightness in a controlled factory environment. This helps to reduce air leakage through the envelope.
In selecting strategies for improving airtightness, it is important to consider the specific climate and building design. In cold climates, it is particularly important to seal air leakage pathways on the warm side of the insulation to prevent moisture condensation. In hot and humid climates, it is important to allow for some degree of drying potential to prevent moisture accumulation. An integrated approach, considering both thermal and moisture management, is crucial for long-term building performance.
Many thanks to our sponsor Focus 360 Energy who helped us prepare this research report.
5. The Impact of Airtightness on Building Performance
5.1 Energy Efficiency
The most direct and widely recognized impact of airtightness is its effect on energy efficiency. Air leakage can account for a significant portion of a building’s heating and cooling loads, particularly in older buildings with poorly sealed envelopes. By reducing air leakage, it is possible to significantly lower energy consumption and reduce utility bills [9]. Improved airtightness directly reduces the amount of energy needed to heat or cool a building, which reduces the strain on HVAC systems, prolongs their lifespan, and decreases operational costs.
Furthermore, airtightness allows for more precise control of the indoor environment, enabling the use of smaller and more efficient HVAC systems. Infiltration and exfiltration contribute to an increased load on the heating and cooling systems, necessitating larger, more powerful units to maintain desired temperatures. A tighter building envelope reduces this demand, allowing for optimized HVAC sizing and performance.
5.2 Indoor Air Quality
While airtightness is essential for energy efficiency, it can also have a positive impact on indoor air quality (IAQ). By reducing uncontrolled air infiltration, airtightness can help to prevent the entry of outdoor pollutants, such as pollen, dust, and vehicle exhaust. However, it is crucial to provide adequate ventilation to ensure that indoor pollutants, such as volatile organic compounds (VOCs) and carbon dioxide, do not accumulate to unhealthy levels [10].
Mechanical ventilation systems, such as heat recovery ventilators (HRVs) and energy recovery ventilators (ERVs), can provide controlled ventilation while minimizing energy losses. These systems exchange stale indoor air with fresh outdoor air, while recovering heat or energy from the exhaust air stream. This ensures that buildings are adequately ventilated without compromising energy efficiency.
5.3 Building Durability
Airtightness also plays a crucial role in building durability. Air leakage can transport moisture into building cavities, leading to condensation, mold growth, and material degradation. By reducing air leakage, it is possible to minimize moisture intrusion and prevent these problems. However, it is important to carefully consider the hygrothermal properties of building materials and assemblies to ensure that moisture can escape from the building envelope when necessary [11].
Uncontrolled air leakage can also lead to thermal bridging, where heat flows through highly conductive materials in the building envelope. This can result in cold spots on interior surfaces, which can lead to condensation and mold growth. By reducing air leakage and minimizing thermal bridging, it is possible to create a more durable and comfortable building.
5.4 Acoustics
While often overlooked, airtightness contributes to improved acoustic performance in buildings. Cracks and gaps in the building envelope act as pathways for sound transmission, allowing exterior noise to penetrate the interior. By sealing these leakage pathways, airtightness can significantly reduce noise pollution from external sources, such as traffic and aircraft, creating a quieter and more peaceful indoor environment [12].
Furthermore, airtightness can also improve the acoustic isolation between adjacent rooms within a building. A tighter building envelope reduces sound transmission between spaces, enhancing privacy and reducing distractions, particularly in residential buildings and office environments.
Many thanks to our sponsor Focus 360 Energy who helped us prepare this research report.
6. Airtightness and Passive House Standards
The Passive House standard is a rigorous energy efficiency standard that requires buildings to achieve extremely low energy consumption and high levels of indoor comfort. Airtightness is a key requirement of the Passive House standard, with a maximum allowable air leakage rate of 0.6 ACH50 [13].
Achieving the Passive House airtightness standard requires careful attention to detail during the design and construction phases. Air barriers must be continuous and well-sealed, and all penetrations and connections must be carefully detailed. Blower door tests are typically performed during construction to identify and address any air leakage problems. Many construction sites also perform a mid-construction blower door test, this allows any issues to be fixed before the completion of construction. This is often much easier and more cost effective than fixing issues after construction is complete.
Buildings that meet the Passive House standard typically have significantly lower energy consumption and better indoor air quality than conventional buildings. They also tend to be more durable and require less maintenance.
Many thanks to our sponsor Focus 360 Energy who helped us prepare this research report.
7. Airtightness in the Context of Sustainable Building Design
While airtightness is primarily associated with energy efficiency, it also has broader implications for sustainable building design. Reducing energy consumption is a key strategy for mitigating climate change, and airtightness is an essential component of energy-efficient buildings. Furthermore, airtightness can contribute to improved indoor air quality, which is important for the health and well-being of building occupants.
However, it is important to consider the embodied carbon of airtightness materials and strategies. Embodied carbon refers to the greenhouse gas emissions associated with the extraction, manufacturing, transportation, and disposal of building materials. Selecting low-embodied carbon materials and strategies can help to minimize the environmental impact of airtightness measures [14]. For example, choosing a liquid applied membrane instead of a thick concrete wall can reduce the embodied carbon significantly.
Life-cycle assessment (LCA) can be used to evaluate the overall environmental impact of different airtightness strategies, considering both operational energy savings and embodied carbon. LCA can help to identify the most sustainable solutions for improving airtightness in buildings.
Many thanks to our sponsor Focus 360 Energy who helped us prepare this research report.
8. Future Trends in Airtightness
The field of airtightness is constantly evolving, with new technologies and strategies being developed to improve building performance. Some of the key trends in airtightness include:
- Smart building envelopes: Smart building envelopes incorporate sensors and actuators to dynamically adjust their properties in response to changing environmental conditions. For example, smart windows can automatically adjust their shading coefficient to optimize solar gain and reduce cooling loads. Smart air barriers can also be developed to modulate air permeability based on occupancy and ventilation needs.
- Data-driven optimization: Building performance data, such as energy consumption and indoor air quality measurements, can be used to optimize airtightness strategies. Data analytics and machine learning algorithms can identify patterns and correlations that can inform the design and operation of airtight building envelopes.
- Advanced materials: New materials are being developed with improved airtightness properties and lower embodied carbon. For example, bio-based air barrier materials are being developed using renewable resources. Aerogels and vacuum insulation panels are also being explored for their potential to provide high levels of insulation and airtightness in thin profiles.
- Integration with BIM: Building Information Modeling (BIM) can be used to improve the design and construction of airtight building envelopes. BIM allows for the creation of a digital model of the building, which can be used to identify potential air leakage pathways and to optimize the placement of air barriers.
These trends suggest that airtightness will continue to play an increasingly important role in the design and construction of high-performance buildings. By embracing these advancements, it is possible to create buildings that are more energy-efficient, durable, and comfortable.
Many thanks to our sponsor Focus 360 Energy who helped us prepare this research report.
9. Conclusion
Airtightness is a critical performance parameter for modern buildings, impacting energy efficiency, indoor air quality, building durability, and overall sustainability. Achieving high levels of airtightness requires a comprehensive understanding of the physics of air leakage, the availability of accurate measurement techniques, and the implementation of effective sealing strategies. The integration of airtightness considerations into the broader framework of sustainable building design is crucial for mitigating climate change and creating healthier, more resilient built environments. By embracing innovation and adopting a holistic approach, the building industry can unlock the full potential of airtightness to create high-performance buildings that meet the challenges of the 21st century.
Many thanks to our sponsor Focus 360 Energy who helped us prepare this research report.
References
[1] Straube, J. F. (2011). High-performance building envelopes: What, why, and how. Building Science Press.
[2] Lstiburek, J. (2008). Builder’s guide to cold climates. Building Science Press.
[3] ASHRAE. (2017). ASHRAE Handbook—Fundamentals. American Society of Heating, Refrigerating and Air-Conditioning Engineers.
[4] ASTM E779-19, Standard Test Method for Determining Air Leakage Rate by Fan Pressurization, ASTM International, West Conshohocken, PA, 2019, www.astm.org.
[5] Snell, J., & Spring, R. T. (2018). Thermal imaging cameras: Theory, applications, and case studies. CRC Press.
[6] TenWolde, A., & Rose, W. B. (1995). Air infiltration and building materials: An overview. Journal of Thermal Envelope and Building Science, 19(1), 75-90.
[7] Burnett, E. F. P. (2005). Air barrier systems for commercial buildings. ASTM International.
[8] U.S. Department of Energy. (2015). Building America solution center: Air sealing. Retrieved from https://basc.pnnl.gov/
[9] Persily, A., & Emmerich, S. J. (2008). Impact of airtightness on residential energy use. Energy and Buildings, 40(11), 2013-2022.
[10] Howard-Reed, C., & Nazaroff, W. W. (2009). Modeling pollutant transport in and out of U.S. residences: Effect of air exchange rates. Atmospheric Environment, 43(29), 4469-4476.
[11] Straube, J. F., & Burnett, E. F. P. (2005). Moisture control handbook: Principles and practices for residential and small commercial buildings. John Wiley & Sons.
[12] Warnock, A. (2010). Acoustics of buildings. Routledge.
[13] Passive House Institute. (2016). Passive House planning package. Retrieved from https://passivehouse.com/
[14] Hammond, G. P., & Jones, C. I. (2011). Embodied energy and carbon in new and refurbished UK housing: Life cycle assessment and comparison. Energy and Buildings, 43(1), 181-190.
So, if we all lived in Passive Houses, would we need to worry about noisy neighbors? Maybe airtightness is the key to world peace and better sleep!