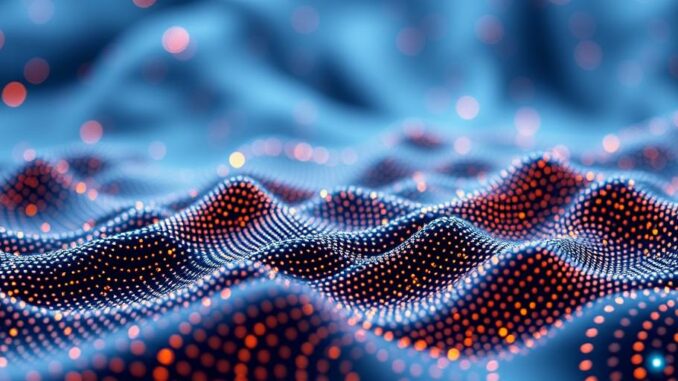
Abstract
This research report explores advanced techniques in acoustic design and measurement for optimizing the acoustic environment within buildings, extending beyond basic Building Research Establishment Environmental Assessment Method (BREEAM) compliance. While BREEAM certification, particularly HEA 05, provides a framework for addressing acoustic comfort, this report delves into cutting-edge methodologies, materials, and psychoacoustic considerations that can significantly enhance occupant well-being and productivity. The report investigates advanced numerical modeling approaches, active noise control (ANC) systems, innovative metamaterials, and sophisticated binaural measurement techniques. Furthermore, it examines the crucial interplay between acoustic design and other environmental factors, such as lighting and ventilation, to achieve a holistic and human-centric built environment. The analysis includes a discussion of the limitations of current acoustic metrics and proposes directions for future research and development in this field.
Many thanks to our sponsor Focus 360 Energy who helped us prepare this research report.
1. Introduction
Acoustics, the science of sound, plays a crucial role in shaping our perception and experience of the built environment. Beyond simply mitigating noise pollution, effective acoustic design can enhance communication clarity, reduce stress levels, improve concentration, and foster a sense of well-being. This is increasingly recognized in building certification schemes such as BREEAM, which awards credits for achieving specific acoustic performance targets. However, a deeper understanding of advanced acoustic principles and technologies can lead to significantly improved outcomes, surpassing the minimum requirements of such certifications.
This report aims to explore these advanced techniques, providing a comprehensive overview of current research and development in acoustic design and measurement. It moves beyond the fundamental principles to examine the complexities of creating optimal acoustic environments in various building types, considering the diverse needs and sensitivities of occupants. The report also addresses the limitations of current standardized metrics and proposes new approaches for evaluating and improving acoustic performance.
Many thanks to our sponsor Focus 360 Energy who helped us prepare this research report.
2. Advanced Numerical Modeling Techniques
Predictive acoustic modeling is essential for informed design decisions. Traditional methods, such as ray tracing and image source methods, offer valuable insights, but they often struggle to accurately simulate complex sound fields, particularly in spaces with intricate geometries or diffuse sound reflections. Advanced numerical techniques, like the Finite Element Method (FEM) and the Boundary Element Method (BEM), provide more accurate and detailed simulations. However, they come with significantly increased computational cost.
-
Finite Element Method (FEM): FEM excels at modeling sound propagation in complex geometries and analyzing the interaction between sound waves and structural elements. It is particularly useful for predicting the acoustic performance of building materials and components, such as walls, floors, and windows. However, FEM simulations can be computationally intensive, especially for large models and high frequencies. Furthermore, correctly defining material properties and boundary conditions for realistic simulations requires detailed information that is not always easily available.
-
Boundary Element Method (BEM): BEM focuses on the boundaries of the acoustic domain, reducing the dimensionality of the problem and often leading to faster computation times compared to FEM for certain types of problems. BEM is well-suited for modeling exterior acoustics and sound radiation from vibrating surfaces. It is particularly effective in predicting sound pressure levels in open spaces and around buildings. A drawback of BEM is its difficulty in modeling inhomogeneous materials and complex internal geometries.
-
Hybrid Methods: To overcome the limitations of individual methods, hybrid approaches combining FEM, BEM, and ray tracing are increasingly employed. For example, FEM can be used to model the structural response of a vibrating panel, BEM can be used to calculate the sound field radiated from the panel, and ray tracing can be used to simulate sound propagation in a large space. These hybrid methods provide a more comprehensive and accurate representation of the acoustic environment.
-
Acoustic Metamaterials Modeling: Acoustic metamaterials, discussed further below, require specialized modeling techniques. Due to their subwavelength features, homogenization techniques are often applied to simplify the simulation. However, full-wave simulations using FEM or Finite-Difference Time-Domain (FDTD) methods are sometimes necessary to capture the intricate wave behavior within these structures.
Advanced modeling software packages incorporate sophisticated algorithms and visualization tools to facilitate the design process. These tools allow architects and engineers to quickly evaluate different design options and identify potential acoustic problems before construction begins. However, the accuracy of these simulations depends critically on the quality of the input data and the expertise of the user.
Many thanks to our sponsor Focus 360 Energy who helped us prepare this research report.
3. Active Noise Control (ANC) Systems
Active Noise Control (ANC) represents a sophisticated approach to noise reduction that utilizes electronic systems to generate anti-noise signals that cancel out unwanted sound. Unlike passive noise control methods that rely on sound absorption and isolation, ANC can be particularly effective at low frequencies, where passive methods are often less efficient and require bulky materials.
-
Fundamentals of ANC: ANC systems typically consist of microphones to detect unwanted noise, a signal processor to generate the anti-noise signal, and loudspeakers to emit the anti-noise signal. The anti-noise signal is designed to be 180 degrees out of phase with the unwanted noise, causing destructive interference and reducing the overall sound level.
-
Types of ANC Systems: ANC systems can be classified into feedforward and feedback systems. Feedforward systems use microphones to detect the noise source before it reaches the target location, allowing for more precise cancellation. Feedback systems, on the other hand, use microphones located at the target location to monitor the residual noise and adjust the anti-noise signal accordingly. Hybrid systems combine both feedforward and feedback control to achieve optimal performance.
-
Applications in Buildings: ANC systems can be implemented in various building applications, including HVAC systems, machinery rooms, and open-plan offices. In HVAC systems, ANC can effectively reduce the noise generated by fans and compressors. In machinery rooms, ANC can mitigate the noise emitted by industrial equipment. In open-plan offices, ANC can create quiet zones by reducing the ambient noise level and improving speech privacy.
-
Challenges and Limitations: The effectiveness of ANC systems depends on several factors, including the characteristics of the noise source, the acoustic environment, and the performance of the electronic components. ANC systems are generally more effective for stationary noise sources and in well-defined acoustic environments. Challenges include the complexity of implementing ANC in reverberant spaces, the potential for instability in feedback systems, and the cost of the electronic components. Careful design and tuning are required to achieve optimal performance.
Many thanks to our sponsor Focus 360 Energy who helped us prepare this research report.
4. Acoustic Metamaterials and Phononic Crystals
Acoustic metamaterials and phononic crystals represent a revolutionary approach to sound manipulation. These artificially engineered structures exhibit acoustic properties not found in nature, enabling unprecedented control over sound waves. They offer the potential to create innovative solutions for noise reduction, sound insulation, and acoustic cloaking.
-
Acoustic Metamaterials: Acoustic metamaterials are typically composed of periodic arrangements of subwavelength structures designed to manipulate sound waves at specific frequencies. These structures can exhibit negative effective mass density or negative effective bulk modulus, leading to unusual acoustic phenomena such as negative refraction and acoustic cloaking. Examples of acoustic metamaterials include coiled space structures, membrane-type metamaterials, and Helmholtz resonator arrays.
-
Phononic Crystals: Phononic crystals are periodic structures with alternating acoustic properties that create band gaps, frequency ranges where sound waves cannot propagate. By carefully designing the geometry and material properties of the phononic crystal, it is possible to create band gaps at desired frequencies, effectively blocking sound transmission. Phononic crystals can be used for sound insulation, vibration isolation, and acoustic filtering.
-
Applications in Building Acoustics: Acoustic metamaterials and phononic crystals have potential applications in building acoustics, including soundproof walls, noise barriers, and vibration dampers. By incorporating these materials into building components, it is possible to achieve significant improvements in sound insulation and noise reduction. However, the cost and scalability of these materials remain a challenge for widespread adoption. Furthermore, the performance of metamaterials is often highly frequency-dependent, requiring careful design to match the specific noise spectrum.
-
Future Directions: Research in acoustic metamaterials and phononic crystals is rapidly evolving. Future directions include the development of tunable metamaterials that can adapt to changing acoustic environments, the creation of broadband metamaterials that operate over a wide frequency range, and the integration of metamaterials with conventional building materials.
Many thanks to our sponsor Focus 360 Energy who helped us prepare this research report.
5. Advanced Binaural Measurement Techniques
Binaural measurements capture the sound field as perceived by a human listener, providing a more realistic and comprehensive assessment of the acoustic environment than traditional sound pressure level measurements. These measurements are obtained using a dummy head or in-ear microphones that mimic the human auditory system.
-
Binaural Recording: Binaural recording involves capturing sound using microphones placed at the ear canals of a dummy head or a human subject. The resulting recordings contain information about the direction, distance, and timbre of sound sources, as well as the effects of head-related transfer functions (HRTFs). These recordings can be played back through headphones to recreate the spatial sound field as perceived by the listener.
-
Head-Related Transfer Functions (HRTFs): HRTFs describe how the sound field is modified by the listener’s head, torso, and pinnae (outer ears). These functions are unique to each individual and depend on the direction and distance of the sound source. HRTFs play a crucial role in spatial hearing, allowing us to localize sound sources in three dimensions.
-
Binaural Room Impulse Responses (BRIRs): BRIRs are impulse responses measured at the ear canals of a dummy head or a human subject in a room. BRIRs contain information about the direct sound, early reflections, and late reverberation of the room. They provide a comprehensive characterization of the acoustic environment and can be used to simulate realistic acoustic experiences.
-
Psychoacoustic Analysis: Binaural measurements can be analyzed using psychoacoustic metrics to evaluate the subjective perception of the acoustic environment. These metrics include loudness, sharpness, roughness, and fluctuation strength. Psychoacoustic analysis can provide valuable insights into the perceived quality of sound and can be used to optimize acoustic design for human comfort and well-being.
-
Applications in Building Acoustics: Binaural measurements are used in various building acoustics applications, including the evaluation of speech intelligibility, the assessment of noise annoyance, and the design of virtual acoustic environments. They provide a powerful tool for understanding how people perceive sound in buildings and for creating acoustic environments that are both functional and aesthetically pleasing.
Many thanks to our sponsor Focus 360 Energy who helped us prepare this research report.
6. Integrating Acoustics with Other Environmental Factors
Effective acoustic design cannot be considered in isolation. The interaction between acoustics and other environmental factors, such as lighting, ventilation, and thermal comfort, is crucial for creating a holistic and human-centric built environment.
-
Acoustics and Lighting: Lighting can influence the perceived loudness of sound and the overall impression of the acoustic environment. For example, bright lighting can make noise seem more intrusive, while dim lighting can create a more relaxed and intimate atmosphere. Integrating acoustic and lighting design can create a more harmonious and comfortable environment. For instance, strategically placed lighting fixtures can also serve as acoustic diffusers, reducing sound reflections and improving speech clarity. Similarly, the surface treatments chosen for acoustic control can also affect light reflectance values, influencing the overall brightness and visual comfort of the space. There is also evidence of physiological links, for example, certain types of flickering lights can exacerbate conditions such as tinnitus.
-
Acoustics and Ventilation: Ventilation systems can be a significant source of noise in buildings. Fans, ducts, and diffusers can generate unwanted sound that can disrupt speech and reduce concentration. Designing ventilation systems with low noise levels is essential for creating a comfortable acoustic environment. Additionally, the placement of ventilation inlets and outlets can affect the sound field in a room, potentially creating areas of high noise concentration. Careful consideration of the acoustic properties of ventilation components and their placement can minimize noise transmission. The use of active noise control in HVAC systems, as discussed earlier, is particularly relevant here.
-
Acoustics and Thermal Comfort: Thermal comfort can also influence the perceived quality of the acoustic environment. Uncomfortable temperatures can increase stress levels and make people more sensitive to noise. Conversely, a comfortable thermal environment can help to reduce stress and improve tolerance to noise. Acoustic materials can also contribute to thermal insulation, helping to regulate temperature and reduce energy consumption. Some studies suggest a correlation between thermal discomfort and increased sensitivity to low-frequency noise.
-
Holistic Design Approach: A holistic design approach that considers the interactions between acoustics and other environmental factors is essential for creating buildings that are both functional and comfortable. This requires close collaboration between architects, engineers, and other building professionals.
Many thanks to our sponsor Focus 360 Energy who helped us prepare this research report.
7. Limitations of Current Acoustic Metrics and Future Directions
While current acoustic metrics, such as reverberation time (RT60), sound pressure level (SPL), and speech transmission index (STI), provide valuable information about the acoustic environment, they have limitations in capturing the subjective perception of sound. These metrics often fail to account for the complex interplay between sound and human cognition.
-
Subjective Perception: Current acoustic metrics primarily focus on objective measurements of sound, neglecting the subjective perception of sound by individual listeners. Factors such as age, hearing ability, and personal preferences can significantly influence how people perceive sound. Future research should focus on developing metrics that incorporate subjective perception and account for individual differences.
-
Psychoacoustic Metrics: Psychoacoustic metrics, such as loudness, sharpness, roughness, and fluctuation strength, offer a more nuanced assessment of the perceived quality of sound. However, these metrics are not yet widely used in building acoustics standards and guidelines. Further research is needed to validate the effectiveness of psychoacoustic metrics in predicting human response to sound in buildings.
-
Acoustic Comfort: Acoustic comfort is a complex and multifaceted concept that encompasses not only the absence of noise but also the presence of pleasant and stimulating sounds. Current acoustic metrics fail to capture the full range of factors that contribute to acoustic comfort. Future research should focus on developing a more comprehensive definition of acoustic comfort and on identifying the factors that influence it.
-
Artificial Intelligence and Machine Learning: Artificial intelligence (AI) and machine learning (ML) techniques offer promising avenues for advancing acoustic design and measurement. AI/ML algorithms can be trained to predict acoustic performance based on design parameters, optimize acoustic treatments, and personalize acoustic environments to individual preferences. These technologies can also be used to analyze large datasets of acoustic measurements and identify patterns that would be difficult to detect using traditional methods. Further research is needed to explore the full potential of AI/ML in building acoustics.
-
Personalized Acoustics: Future acoustic environments will likely be personalized to the individual needs and preferences of occupants. This could involve using wearable devices to monitor physiological responses to sound and adjust acoustic parameters in real-time. Personalized acoustics could also involve creating virtual acoustic environments that are tailored to the individual’s task or activity.
Many thanks to our sponsor Focus 360 Energy who helped us prepare this research report.
8. Conclusion
Advanced acoustic design and measurement techniques offer significant opportunities for enhancing the built environment. By leveraging sophisticated numerical modeling, active noise control, acoustic metamaterials, and binaural measurement techniques, it is possible to create spaces that are not only quieter but also more comfortable, productive, and healthy. While current acoustic metrics provide a valuable foundation, future research should focus on incorporating subjective perception, developing psychoacoustic metrics, and exploring the potential of artificial intelligence and personalized acoustics. By adopting a holistic design approach that integrates acoustics with other environmental factors, we can create buildings that truly enhance the well-being of their occupants.
Many thanks to our sponsor Focus 360 Energy who helped us prepare this research report.
References
- Beranek, L. L., & Mellow, T. J. (2012). Acoustics: Sound Fields and Transducers. Academic Press.
- Cox, T. J., & D’Antonio, P. (2017). Acoustic Absorbers and Diffusers: Theory, Design and Application. CRC press.
- Kinsler, L. E., Frey, A. R., Coppens, A. B., & Sanders, J. V. (2000). Fundamentals of Acoustics. John Wiley & Sons.
- Pierce, A. D. (1989). Acoustics: An Introduction to Its Physical Principles and Applications. Acoustical Society of America.
- Bistafa, S. R., (2011). Acoustic measurements. Academic Press.
- Fahy, F. J., & Gardonio, P. (2007). Sound and structural vibration: radiation, transmission and response. Academic Press.
- Nelson, P. A., & Elliott, S. J. (1992). Active Control of Sound. Academic Press.
- Fang, N., Xi, D., Xu, J., Ambati, M., Sun, W., & Zhang, X. (2006). Ultrasonic metamaterials with negative modulus. Nature Materials, 5(6), 452-456.
- Yang, Z., Jun Mei, X., Yang, M., Chan, N. H., & Sheng, P. (2008). Membrane-type acoustic metamaterial with negative dynamic mass. Physical Review Letters, 101(20), 204301.
- Blauert, J. (1997). Spatial Hearing: The Psychophysics of Human Sound Localization. MIT Press.
- Møller, H., Sørensen, M. F., Hammershøi, D., & Jensen, C. B. (2006). Binaural technique: Do we measure headphones as accurately as we think?. Journal of the Audio Engineering Society, 54(3), 203-223.
- ISO 3382-1:2009. Acoustics — Measurement of room acoustic parameters — Part 1: Performance spaces.
- ISO 16283-1:2014. Acoustics — Field measurement of sound insulation in buildings and of building elements — Part 1: Airborne sound insulation.
- BREEAM UK New Construction. SD5073:2018 (Version 6.0). BRE Global Ltd.
The discussion on integrating acoustics with lighting and ventilation is particularly insightful. Has anyone explored the impact of biophilic design elements, such as indoor plants or water features, on perceived acoustic comfort and overall well-being in built environments?
That’s a fantastic point! We touched on the interplay of environmental factors, and biophilic elements are definitely worth exploring further. There is a growing body of research suggesting that these elements can reduce stress. I wonder if anyone has quantitative data on how things like water features affect acoustic absorption or diffusion?
Editor: FocusNews.Uk
Thank you to our Sponsor Focus 360 Energy
Given the increasing use of hybrid methods in numerical modeling, what are your thoughts on the accessibility and training resources available for practitioners to effectively utilize these complex simulation tools for acoustic design?
That’s a great question! While hybrid modeling offers fantastic potential, the learning curve can be steep. Increased accessibility hinges on more comprehensive training programs and user-friendly interfaces for these tools. Perhaps online platforms could provide specialized courses and collaborative spaces to share best practices and overcome challenges in applying these advanced methods. What resources have you found most helpful?
Editor: FocusNews.Uk
Thank you to our Sponsor Focus 360 Energy
This report highlights the importance of psychoacoustic metrics. Could further research explore how individual HRTFs impact the design of personalized acoustic environments, moving beyond standardized dummy head measurements?
That’s a great point about individual HRTFs! Personalized acoustic environments are definitely the future. Exploring how we can tailor designs based on individual hearing profiles, going beyond the standard dummy head measurements, could significantly improve user experience and comfort. It would be great to see research in this area. Does anyone have resources or experience in individual HRTF data collection?
Editor: FocusNews.Uk
Thank you to our Sponsor Focus 360 Energy