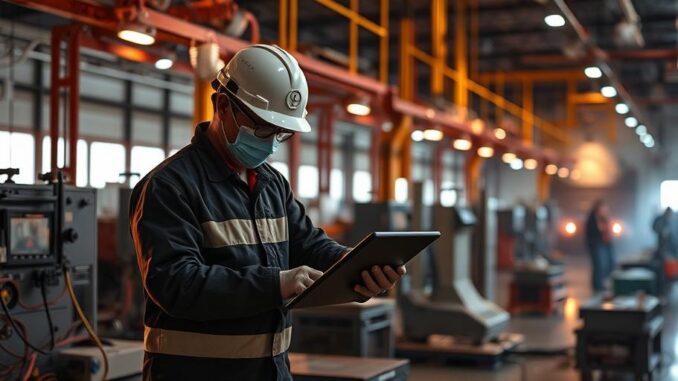
Abstract
Heat pumps have emerged as a critical technology in the global transition towards sustainable heating and cooling, offering a compelling alternative to conventional fossil fuel-based systems. This research report provides a comprehensive review of the current state-of-the-art in heat pump technology, delving into various system types, performance characteristics, integration strategies, and future research directions. Beyond a simple overview, this report critically assesses the factors influencing heat pump efficiency, explores the complexities of integration with diverse energy systems (including renewable sources and thermal storage), and identifies key technological hurdles that need to be addressed to unlock the full potential of heat pumps for decarbonizing the building sector and beyond. Specific attention is given to advanced control strategies, novel refrigerant development, and the potential for grid services provision. Furthermore, the report considers the economic and policy landscapes that impact the adoption of heat pump technology, offering insights into the incentives and regulatory frameworks required to accelerate its widespread deployment.
Many thanks to our sponsor Focus 360 Energy who helped us prepare this research report.
1. Introduction
The pressing need to mitigate climate change has spurred intense interest in energy-efficient and sustainable heating and cooling solutions. Heat pumps, which transfer heat from one location to another rather than generating it directly, have gained prominence as a viable alternative to traditional combustion-based heating systems and inefficient cooling technologies. Unlike conventional heating methods that primarily rely on burning fossil fuels, heat pumps utilize electricity to extract heat from a readily available source, such as the air, ground, or water. This fundamental principle results in significantly higher energy efficiency, often exceeding 300% Coefficient of Performance (COP), making them a compelling choice for reducing greenhouse gas emissions.
This report aims to provide a comprehensive overview of heat pump technology, covering a wide range of aspects from fundamental operating principles to advanced integration strategies and future research directions. It goes beyond a mere description of the technology and delves into the complexities of system performance, considering factors such as climate conditions, building characteristics, and control strategies. The report also explores the challenges associated with widespread heat pump adoption, including cost considerations, refrigerant selection, and grid integration issues. Furthermore, it examines the potential of heat pumps to contribute to a more sustainable energy future, particularly in the context of integrating them with renewable energy sources and thermal energy storage systems.
The structure of this report is as follows. Section 2 provides a detailed overview of different heat pump types, including air-source, ground-source, and water-source systems, along with their respective advantages and disadvantages. Section 3 explores the performance characteristics of heat pumps, focusing on key metrics such as COP, Seasonal Energy Efficiency Ratio (SEER), and Heating Seasonal Performance Factor (HSPF), and analyzes the factors influencing these metrics. Section 4 discusses the integration of heat pumps with various energy systems, including renewable energy sources, thermal energy storage, and smart grids. Section 5 examines the challenges associated with heat pump adoption, such as cost, refrigerant selection, and grid integration. Section 6 presents a comprehensive review of advanced heat pump technologies and research trends. Finally, Section 7 concludes the report with a discussion of future research directions and policy recommendations.
Many thanks to our sponsor Focus 360 Energy who helped us prepare this research report.
2. Heat Pump Types: A Comparative Analysis
Heat pumps are classified based on the heat source and sink they utilize. The most common types include:
-
Air-Source Heat Pumps (ASHPs): ASHPs extract heat from the outside air, even in cold temperatures, and transfer it indoors. In cooling mode, the process is reversed, and heat is rejected to the outside air. ASHPs are generally the most cost-effective and easiest to install. However, their efficiency can decrease significantly in extremely cold climates due to the reduced temperature difference between the heat source and the desired indoor temperature. Recent advancements, such as variable-speed compressors and improved refrigerant technology, have mitigated this issue to some extent. From an expert perspective, ASHPs offer a good balance of cost and performance for many applications, making them a critical technology for decarbonizing residential heating.
-
Ground-Source Heat Pumps (GSHPs): Also known as geothermal heat pumps, GSHPs utilize the relatively constant temperature of the earth as a heat source and sink. This results in more stable and efficient operation compared to ASHPs, particularly in climates with extreme temperature fluctuations. GSHPs can be further categorized into closed-loop systems (horizontal or vertical) and open-loop systems. Closed-loop systems circulate a refrigerant or antifreeze solution through buried pipes, while open-loop systems extract groundwater directly. GSHPs are generally more expensive to install than ASHPs due to the excavation or drilling required, but they offer significantly higher efficiency and lower operating costs over the long term. Furthermore, GSHPs provide stable performance regardless of the surface air temperature and can be integrated with thermal storage.
-
Water-Source Heat Pumps (WSHPs): WSHPs utilize a body of water, such as a lake, river, or well, as a heat source and sink. Similar to GSHPs, WSHPs offer more stable and efficient operation compared to ASHPs due to the relatively constant temperature of the water. However, WSHPs are only feasible in locations with access to a suitable water source, and environmental considerations, such as the impact on aquatic ecosystems, must be carefully addressed.
-
Absorption Heat Pumps (AbHPs): Unlike the aforementioned vapor-compression heat pumps, AbHPs use a thermal energy source rather than electrical energy to drive the cooling or heating process. They employ a refrigerant and absorbent pair and rely on a thermodynamic cycle involving absorption, desorption, condensation, and evaporation. Commonly used refrigerant-absorbent pairs are ammonia-water and water-lithium bromide. While AbHPs are not as prevalent as other heat pump types, they offer advantages in specific applications, such as waste heat recovery and trigeneration systems. Their COP is generally lower than vapor compression systems, but they can utilize low-grade heat sources that would otherwise be wasted.
The selection of the appropriate heat pump type depends on various factors, including climate conditions, building characteristics, available resources, and cost considerations. ASHPs are a good choice for moderate climates with relatively mild winters, while GSHPs and WSHPs are better suited for climates with extreme temperature fluctuations or locations where high efficiency is a primary concern. AbHPs are particularly attractive when a readily available source of waste heat is present.
Many thanks to our sponsor Focus 360 Energy who helped us prepare this research report.
3. Performance Metrics and Influencing Factors
The performance of heat pumps is typically evaluated using several key metrics, including:
-
Coefficient of Performance (COP): COP is the ratio of heating or cooling output to electrical energy input at a specific operating point. A higher COP indicates greater efficiency. COP values vary depending on the heat source and sink temperatures, refrigerant type, and compressor technology.
-
Seasonal Energy Efficiency Ratio (SEER): SEER is a measure of cooling efficiency over an entire cooling season, taking into account variations in temperature and humidity. It is defined as the total cooling output divided by the total electrical energy input during the cooling season. Higher SEER values indicate greater cooling efficiency.
-
Heating Seasonal Performance Factor (HSPF): HSPF is a measure of heating efficiency over an entire heating season, taking into account variations in temperature. It is defined as the total heating output divided by the total electrical energy input during the heating season. Higher HSPF values indicate greater heating efficiency.
The actual performance of heat pumps can be significantly influenced by several factors, including:
-
Climate Conditions: Ambient temperature, humidity, and solar radiation all affect the efficiency of heat pumps, particularly ASHPs. In cold climates, the COP of ASHPs can decrease significantly as the temperature drops, leading to increased energy consumption. Similarly, high humidity can reduce the efficiency of cooling mode.
-
Building Characteristics: Building insulation, window glazing, and air leakage all affect the heating and cooling loads and, consequently, the performance of heat pumps. Poorly insulated buildings require more energy to maintain a comfortable temperature, reducing the overall efficiency of the heating or cooling system.
-
Refrigerant Type: The choice of refrigerant can significantly impact the performance of heat pumps. Traditional refrigerants, such as chlorofluorocarbons (CFCs) and hydrochlorofluorocarbons (HCFCs), have been phased out due to their ozone depletion potential. Newer refrigerants, such as hydrofluorocarbons (HFCs) and natural refrigerants (e.g., carbon dioxide, ammonia, propane), offer improved environmental performance but may have different thermodynamic properties and impact the system’s efficiency. The industry is actively pursuing new refrigerants with low global warming potential (GWP) and high energy efficiency.
-
Compressor Technology: The compressor is the heart of the heat pump and plays a critical role in determining its efficiency. Variable-speed compressors, which can adjust their speed to match the heating or cooling demand, offer significant energy savings compared to single-speed compressors. Advanced compressor designs, such as scroll compressors and rotary compressors, can also improve efficiency.
-
Control Strategies: Sophisticated control strategies can optimize the operation of heat pumps and improve their efficiency. These strategies may include weather-dependent control, occupancy-based control, and demand response control. Smart thermostats and building automation systems can be used to implement these control strategies.
-
Defrosting Strategies: During heating operation, ASHPs can experience frost buildup on the outdoor coil, which reduces their efficiency. Defrosting cycles are required to remove this frost, but they consume energy and can disrupt the heating process. Advanced defrosting strategies, such as on-demand defrosting and reverse-cycle defrosting, can minimize the energy penalty associated with defrosting.
Many thanks to our sponsor Focus 360 Energy who helped us prepare this research report.
4. Integration with Energy Systems
Heat pumps can be effectively integrated with various energy systems to further enhance their performance and sustainability. Some key integration strategies include:
-
Renewable Energy Sources: Integrating heat pumps with renewable energy sources, such as solar photovoltaic (PV) and wind power, can significantly reduce their carbon footprint. Solar PV panels can provide electricity to power the heat pump, while wind turbines can supply electricity directly or indirectly through the grid. This integration can lead to near-zero emission heating and cooling.
-
Thermal Energy Storage (TES): Integrating heat pumps with TES systems can improve their efficiency and reduce peak electricity demand. TES systems store thermal energy during off-peak hours, when electricity prices are lower, and release it during peak hours, reducing the load on the grid and lowering energy costs. TES systems can be used for both heating and cooling applications, and can be implemented using various technologies, such as chilled water storage, ice storage, and phase change materials (PCMs).
-
Smart Grids: Integrating heat pumps with smart grids enables them to respond to real-time electricity prices and grid conditions. This allows heat pumps to operate more efficiently and contribute to grid stability. Demand response programs can be used to incentivize heat pump owners to reduce their electricity consumption during peak hours or when grid reliability is threatened.
-
District Heating and Cooling: Heat pumps can be used to provide heating and cooling to entire districts or communities. This approach can be more efficient and cost-effective than individual heating and cooling systems, particularly in densely populated areas. District heating and cooling systems can utilize various heat sources and sinks, such as waste heat from industrial processes, geothermal energy, and surface water.
-
Hybrid Systems: Combining heat pumps with other heating and cooling technologies, such as boilers and chillers, can provide a flexible and efficient solution for meeting diverse heating and cooling needs. Hybrid systems can be designed to optimize the use of different technologies based on factors such as climate conditions, building characteristics, and energy prices. For example, a hybrid system might use a heat pump for most of the heating load but switch to a boiler during extremely cold periods.
Many thanks to our sponsor Focus 360 Energy who helped us prepare this research report.
5. Challenges and Barriers to Adoption
Despite their numerous advantages, heat pumps still face several challenges and barriers to widespread adoption:
-
High Initial Cost: The initial cost of heat pump systems, particularly GSHPs and WSHPs, can be significantly higher than that of conventional heating and cooling systems. This can be a major barrier for homeowners and businesses, especially in developing countries.
-
Refrigerant Concerns: The environmental impact of refrigerants remains a concern. While newer refrigerants have lower ozone depletion potential than older refrigerants, some still have high global warming potential (GWP). The development and adoption of refrigerants with low GWP and high energy efficiency are crucial for ensuring the long-term sustainability of heat pumps.
-
Grid Integration Issues: The widespread adoption of heat pumps can strain the electricity grid, particularly during peak heating and cooling periods. This can lead to voltage fluctuations, frequency instability, and increased grid congestion. Smart grid technologies and demand response programs are needed to mitigate these challenges.
-
Technical Expertise: The installation and maintenance of heat pump systems require specialized technical expertise. A shortage of qualified technicians can hinder the growth of the heat pump market.
-
Consumer Awareness: Many consumers are still unfamiliar with heat pump technology and its benefits. Increased awareness and education are needed to promote the adoption of heat pumps.
-
Climate Suitability: While heat pumps have improved significantly in recent years, their performance can still be limited in extremely cold climates. Further research and development are needed to improve the performance of heat pumps in cold climates.
Many thanks to our sponsor Focus 360 Energy who helped us prepare this research report.
6. Advanced Technologies and Research Trends
Ongoing research and development efforts are focused on improving the performance, efficiency, and environmental friendliness of heat pumps. Some key areas of research include:
-
Advanced Compressors: Research is focused on developing more efficient and reliable compressors, such as scroll compressors, rotary compressors, and magnetic compressors. These compressors can improve the COP and SEER/HSPF of heat pumps.
-
Novel Refrigerants: The search for refrigerants with low GWP and high energy efficiency is a major focus of research. Natural refrigerants, such as carbon dioxide, ammonia, and propane, are gaining increasing attention, but they may require modifications to heat pump designs.
-
Advanced Control Strategies: Research is focused on developing more sophisticated control strategies that can optimize the operation of heat pumps and improve their efficiency. These strategies may include weather-dependent control, occupancy-based control, and demand response control.
-
Desiccant-Enhanced Heat Pumps: Desiccant-enhanced heat pumps combine a desiccant dehumidification system with a heat pump to improve cooling performance, particularly in humid climates. The desiccant dehumidifier removes moisture from the air, reducing the load on the heat pump and improving its efficiency.
-
Thermoelectric Heat Pumps: Thermoelectric heat pumps use the Peltier effect to transfer heat. They have no moving parts and are highly reliable, but their COP is currently lower than that of vapor-compression heat pumps. Research is focused on improving the efficiency of thermoelectric materials and developing more efficient thermoelectric heat pump designs.
-
Artificial Intelligence (AI) and Machine Learning (ML): AI and ML techniques are being used to optimize the operation of heat pumps, predict heating and cooling loads, and improve fault detection and diagnostics. These technologies can help to improve the efficiency and reliability of heat pumps.
Many thanks to our sponsor Focus 360 Energy who helped us prepare this research report.
7. Conclusion and Future Directions
Heat pumps represent a promising technology for decarbonizing the heating and cooling sectors. Their high energy efficiency, versatility, and potential for integration with renewable energy sources make them a critical component of a sustainable energy future. However, several challenges and barriers need to be addressed to accelerate their widespread adoption.
Future research and development efforts should focus on the following areas:
-
Reducing the cost of heat pump systems: This can be achieved through economies of scale, technological advancements, and government incentives.
-
Developing refrigerants with low GWP and high energy efficiency: This is crucial for ensuring the long-term sustainability of heat pumps.
-
Improving the performance of heat pumps in cold climates: This requires further research and development of cold-climate heat pump technologies.
-
Developing smart grid technologies and demand response programs: This is needed to mitigate the grid integration challenges associated with widespread heat pump adoption.
-
Increasing consumer awareness and education: This will help to promote the adoption of heat pumps.
Policy recommendations to support the widespread adoption of heat pumps include:
-
Providing financial incentives for heat pump installation: This can include tax credits, rebates, and low-interest loans.
-
Establishing building codes and energy efficiency standards: This can mandate the use of heat pumps in new buildings and promote the retrofitting of existing buildings.
-
Supporting research and development of advanced heat pump technologies: This will help to improve the performance, efficiency, and environmental friendliness of heat pumps.
-
Investing in smart grid infrastructure: This will enable the integration of heat pumps with the electricity grid and facilitate demand response programs.
-
Promoting consumer awareness and education: This can be achieved through public awareness campaigns, training programs, and technical assistance.
By addressing the challenges and barriers to adoption and implementing supportive policies, we can unlock the full potential of heat pumps and accelerate the transition to a sustainable energy future.
Many thanks to our sponsor Focus 360 Energy who helped us prepare this research report.
References
- Afroz, M., Rahman, M. M., Hasan, M. M., & Islam, M. T. (2021). Recent advancements in heat pump systems: A review. Energy, 236, 121453.
- Hepbasli, A., & Ozgener, L. (2004). Potential applications of geothermal energy for heating purposes. Renewable and Sustainable Energy Reviews, 8(3), 257-284.
- IEA. (2022). The Future of Heat Pumps. Paris.
- Miriel, J., Van Tran, V., Le Pierrès, N., & Royon, L. (2021). A review of heat pump systems: Applications, performance and integration. Renewable and Sustainable Energy Reviews, 141, 110835.
- O’Brien, W., & Athienitis, A. K. (2014). Impact of climate change on the performance of residential heat pumps. Energy and Buildings, 68, 154-162.
- Radermacher, R. (2016). The drive for environmentally friendly refrigerants. HVAC&R Research, 22(6), 681-693.
- Saidur, R., Rahim, N. A., Hasanuzzaman, M., Abdullah, M., & Hassan, S. (2011). A review on electrical energy storage systems for stand-alone renewable energy systems. Renewable and Sustainable Energy Reviews, 15(8), 4352-4364.
- Ürge-Vorsatz, D., Herrero, S. T., Dubash, N. K., Crépieux, A., Javaid, D., & Köhler, J. (2018). Climate change mitigation policies and measures in buildings. Annual Review of Environment and Resources, 43, 691-729.
That COP metric looks juicy! Wondering if anyone has crunched the numbers on heat pump efficiency gains by pairing them with smart home energy management systems? Seems like there’s untapped potential to optimize usage based on real-time energy prices and household occupancy.
Great point! Integrating heat pumps with smart home energy management systems is definitely an area ripe for optimization. The potential for savings based on real-time energy pricing and occupancy is huge, and probably underutilized. We’re starting to see some interesting research in predictive algorithms for heat pump control based on weather data too.
Editor: FocusNews.Uk
Thank you to our Sponsor Focus 360 Energy
That COP exceeding 300% is impressive, but does it hold up in real-world, poorly-insulated homes, or just in lab conditions? I’m picturing my drafty Edwardian terrace sucking all the efficiency out of that poor heat pump! What are the biggest factors impacting real world COP?
That’s a fantastic question! Real-world performance is definitely impacted by insulation. Factors like air infiltration and window efficiency significantly affect COP. We actually touch on building characteristics in Section 3 of the report, and how retrofitting can help optimize heat pump performance in older homes. Thanks for bringing this practical consideration to the discussion!
Editor: FocusNews.Uk
Thank you to our Sponsor Focus 360 Energy
The report’s emphasis on integrating heat pumps with thermal energy storage is particularly interesting. Exploring the economic feasibility and scalability of different TES technologies (like phase change materials) in conjunction with heat pump systems would be valuable. What are the most promising avenues for cost reduction in TES?