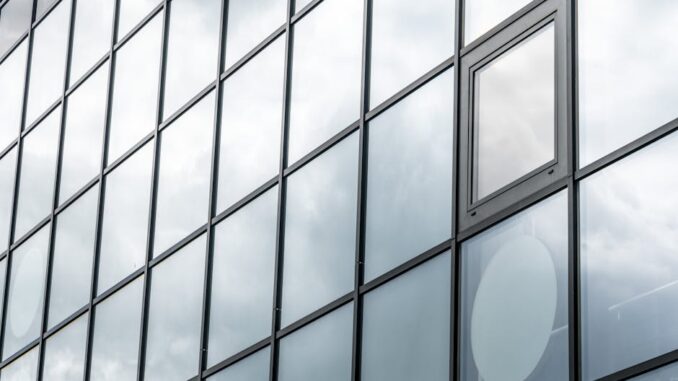
Abstract
Fenestration systems, primarily windows, represent a significant source of energy loss in buildings, particularly in older structures and regions with extreme climates. This research report provides a comprehensive analysis of advanced fenestration technologies aimed at mitigating this energy drain. It examines the underlying physics of heat transfer through windows, evaluates the effectiveness of various energy-efficient technologies, including multi-pane glazing, low-emissivity (low-E) coatings, gas fills, insulated frames, and dynamic glazing systems. Furthermore, the report delves into the life-cycle cost analysis of window upgrades, considering regional climate variations and building characteristics. Emerging trends in fenestration design and performance, such as vacuum insulated glazing (VIG) and advanced shading strategies, are also explored. This analysis aims to provide a critical assessment of current and future technologies to inform building design professionals, policymakers, and homeowners in making informed decisions regarding energy-efficient fenestration.
Many thanks to our sponsor Focus 360 Energy who helped us prepare this research report.
1. Introduction
The building sector is a major consumer of energy globally, accounting for a significant portion of greenhouse gas emissions. A substantial part of this energy consumption is attributed to space heating and cooling, where fenestration systems play a crucial role. Windows, in particular, are often the weakest link in a building’s thermal envelope, acting as a primary pathway for heat transfer between the interior and exterior environments. Older, single-pane windows, prevalent in many existing buildings, offer minimal resistance to heat flow, leading to substantial energy losses during both heating and cooling seasons.
The development and implementation of energy-efficient fenestration technologies are therefore critical for reducing building energy consumption and mitigating its environmental impact. This research report aims to provide a comprehensive overview of these technologies, examining their underlying principles, performance characteristics, and economic viability. The focus is on both established technologies, such as double and triple glazing with low-E coatings and gas fills, and emerging technologies that hold promise for further improving window energy performance.
The research will consider the following key aspects:
- Fundamentals of Heat Transfer: A review of the mechanisms of heat transfer through windows, including conduction, convection, and radiation.
- Energy-Efficient Window Technologies: A detailed analysis of various technologies designed to reduce heat transfer through windows, including multi-pane glazing, low-E coatings, gas fills, insulated frames, and dynamic glazing systems.
- Life-Cycle Cost Analysis: An evaluation of the economic benefits of window upgrades, considering the initial cost, energy savings, and maintenance expenses over the lifetime of the window.
- Regional Climate Considerations: An examination of how the optimal window selection varies depending on the local climate and building characteristics.
- Emerging Trends: An exploration of emerging technologies and design strategies that hold promise for further improving window energy performance.
Many thanks to our sponsor Focus 360 Energy who helped us prepare this research report.
2. Fundamentals of Heat Transfer Through Windows
Understanding the fundamental principles of heat transfer is essential for evaluating the performance of different window technologies. Heat transfer through windows occurs primarily through three mechanisms: conduction, convection, and radiation.
- Conduction: Conduction is the transfer of heat through a material due to a temperature difference. In windows, heat is conducted through the glass panes, frame, and spacers. The rate of heat conduction is proportional to the thermal conductivity of the material and the temperature difference across it.
- Convection: Convection is the transfer of heat through the movement of fluids (liquids or gases). In windows, convection occurs within the air space between the glass panes and on the interior and exterior surfaces of the window. Warm air rises, creating a convective loop that transfers heat from the warmer surface to the cooler surface.
- Radiation: Radiation is the transfer of heat through electromagnetic waves. All objects emit thermal radiation, with the amount and wavelength of radiation depending on the object’s temperature and emissivity. In windows, radiation is exchanged between the glass panes and between the interior and exterior environments. Solar radiation is a major source of heat gain through windows, while infrared radiation is a mechanism for heat loss.
The overall rate of heat transfer through a window is quantified by its U-factor (or U-value), which represents the amount of heat that flows through one square foot of the window per hour for each degree Fahrenheit (or Celsius) difference between the inside and outside temperatures. A lower U-factor indicates better insulation and reduced heat transfer. SHGC (Solar Heat Gain Coefficient) measures the fraction of solar radiation admitted through a window, both directly transmitted, and absorbed and subsequently released inward. Visible Transmittance (VT) quantifies the amount of visible light that passes through the window.
The relative importance of each heat transfer mechanism depends on the specific window design and operating conditions. For example, in single-pane windows, conduction and convection are the dominant modes of heat transfer. In multi-pane windows, the air space between the panes reduces conductive and convective heat transfer, making radiation more significant. Furthermore, the use of low-E coatings can significantly reduce radiative heat transfer.
Many thanks to our sponsor Focus 360 Energy who helped us prepare this research report.
3. Energy-Efficient Window Technologies
Several technologies have been developed to reduce heat transfer through windows, improving their energy performance. These technologies can be broadly categorized into the following:
3.1 Multi-Pane Glazing
Multi-pane glazing involves using two or more panes of glass separated by an air or gas-filled space. This configuration significantly reduces heat transfer compared to single-pane windows. The air or gas-filled space acts as an insulator, reducing conductive and convective heat transfer. Double-pane windows are a common upgrade from single-pane, offering a substantial improvement in energy efficiency. Triple-pane windows provide even greater insulation, reducing heat transfer further. However, increasing the number of panes also increases the weight and cost of the window. The space between panes is important; if it is too narrow, conduction increases. If it is too wide, convection increases.
3.2 Low-E Coatings
Low-E (low-emissivity) coatings are thin, transparent coatings applied to the surface of the glass that reduce radiative heat transfer. These coatings selectively reflect infrared radiation, which is a major component of heat transfer in windows. Low-E coatings are typically applied to the inner surface of the outermost pane in a double-pane window. There are two main types of low-E coatings: hard coat (pyrolytic) and soft coat (sputtered). Hard coat low-E coatings are more durable but have lower performance than soft coat coatings. Soft coat low-E coatings offer superior performance but are more susceptible to damage and must be protected within the sealed glazing unit. The choice of which Low-E coating to use depends on the window application. In colder climates, high solar heat gain Low-E coatings may be preferred to capture passive solar gains, whereas in warmer climates, Low-E coatings that block solar heat gain may be more beneficial.
3.3 Gas Fills
Gas fills are used to replace the air in the space between the glass panes in multi-pane windows. Gases such as argon and krypton have lower thermal conductivity than air, further reducing conductive and convective heat transfer. Argon is a relatively inexpensive and readily available gas, making it a common choice for gas fills. Krypton has even lower thermal conductivity than argon but is more expensive. The effectiveness of gas fills depends on the width of the air space. If the air space is too narrow, the gas fill will have little effect. If the air space is too wide, convection currents will develop, reducing the effectiveness of the gas fill. The benefit of gas fills is largely lost if the window seal degrades and the gas leaks out, so high quality seals are important.
3.4 Insulated Frames
The window frame can also contribute to heat transfer. Traditional aluminum frames are highly conductive and can act as a thermal bridge, allowing heat to flow easily through the window. Insulated frames are designed to reduce this heat transfer. Common materials for insulated frames include wood, vinyl, fiberglass, and aluminum with thermal breaks. Wood frames offer good insulation but require regular maintenance. Vinyl frames are low-maintenance and relatively inexpensive, but they can expand and contract with temperature changes. Fiberglass frames are strong, durable, and offer good insulation. Aluminum frames with thermal breaks incorporate a non-conductive material between the interior and exterior aluminum components, reducing heat transfer.
3.5 Dynamic Glazing Systems
Dynamic glazing systems, such as electrochromic windows, change their optical properties in response to an external stimulus, such as voltage, light, or temperature. Electrochromic windows can switch between a clear state and a tinted state, reducing solar heat gain and glare. This allows for dynamic control of the window’s energy performance, adapting to changing weather conditions and occupancy patterns. Other dynamic glazing technologies include thermochromic and photochromic windows. Thermochromic windows change their optical properties in response to temperature, while photochromic windows change their optical properties in response to light. While dynamic glazing systems offer great potential for energy savings and improved occupant comfort, they are typically more expensive than static glazing systems.
3.6 Window Attachments
External and internal window attachments can also improve the energy performance of a window. Shutters provide an additional layer of insulation and can reduce heat loss during the winter and heat gain during the summer. Shades and blinds can block solar radiation, reducing heat gain and glare. Films can be applied to windows to reduce solar heat gain, ultraviolet radiation, and glare. The effectiveness of window attachments depends on their material, color, and operation. External window attachments are generally more effective than internal attachments at reducing heat gain. Window films are a cost-effective way to upgrade existing windows, but they can reduce visible light transmittance.
Many thanks to our sponsor Focus 360 Energy who helped us prepare this research report.
4. Life-Cycle Cost Analysis of Window Upgrades
A comprehensive life-cycle cost analysis (LCCA) is essential for evaluating the economic viability of window upgrades. LCCA considers the total cost of ownership over the lifetime of the window, including the initial cost, energy savings, maintenance expenses, and replacement costs. The LCCA should also account for regional climate variations and building characteristics. The initial cost of window upgrades can be significant, especially for high-performance windows. However, the energy savings over the lifetime of the window can offset this initial cost. Maintenance expenses can include cleaning, repairs, and resealing. Replacement costs should be considered for windows with a limited lifespan.
To perform an LCCA, several factors need to be considered:
- Initial Cost: This includes the cost of the windows themselves, as well as the cost of installation. The initial cost can vary significantly depending on the type of window, size, and installation complexity.
- Energy Savings: This is the reduction in energy consumption resulting from the window upgrade. Energy savings can be estimated using energy modeling software or by analyzing historical energy consumption data. The energy savings will depend on the climate, building characteristics, and window performance.
- Maintenance Expenses: This includes the cost of cleaning, repairs, and resealing. Maintenance expenses can vary depending on the type of window and its exposure to the elements.
- Replacement Costs: This is the cost of replacing the window at the end of its lifespan. The lifespan of a window can vary depending on the material, construction, and environmental conditions.
- Discount Rate: This is the rate used to discount future costs and savings to their present value. The discount rate reflects the time value of money and the opportunity cost of capital.
The net present value (NPV) of the window upgrade can be calculated by summing the present value of all costs and savings over the lifetime of the window. A positive NPV indicates that the window upgrade is economically viable. The payback period is the time it takes for the cumulative energy savings to equal the initial cost of the window upgrade. A shorter payback period is generally preferred.
Regional climate variations play a significant role in the LCCA of window upgrades. In colder climates, windows with low U-factors are more beneficial, as they reduce heat loss and lower heating costs. In warmer climates, windows with low SHGCs are more beneficial, as they reduce solar heat gain and lower cooling costs. Building characteristics, such as the size and orientation of the windows, also affect the energy savings potential.
Many thanks to our sponsor Focus 360 Energy who helped us prepare this research report.
5. Regional Climate Considerations
The optimal window selection is highly dependent on the regional climate. Different climates have different heating and cooling demands, and the window should be selected to minimize energy consumption for the specific climate. In cold climates, the primary goal is to minimize heat loss through the windows. Windows with low U-factors and high solar heat gain coefficients (SHGC) are preferred. Low U-factors reduce heat loss, while high SHGCs allow for passive solar heating, reducing heating costs. In warm climates, the primary goal is to minimize solar heat gain. Windows with low SHGCs are preferred. Low SHGCs reduce solar heat gain, minimizing cooling loads and reducing cooling costs. In mixed climates, a balance between heat loss and solar heat gain is needed. Windows with moderate U-factors and SHGCs may be preferred. Dynamic glazing systems can also be beneficial in mixed climates, as they can adjust their optical properties to optimize energy performance for different weather conditions.
The orientation of the windows also affects the optimal window selection. South-facing windows receive the most solar radiation during the winter, making them ideal for passive solar heating in cold climates. East- and west-facing windows receive the most solar radiation during the summer, which can lead to overheating. Shading devices, such as overhangs or awnings, can be used to reduce solar heat gain through east- and west-facing windows.
Climate zone maps, such as those published by the U.S. Department of Energy, can be used to guide window selection. These maps divide the country into different climate zones based on temperature, humidity, and solar radiation. Each climate zone has specific recommendations for window U-factors and SHGCs. Building codes and standards, such as the International Energy Conservation Code (IECC), also specify minimum window performance requirements for different climate zones.
Many thanks to our sponsor Focus 360 Energy who helped us prepare this research report.
6. Emerging Trends in Fenestration Design and Performance
Several emerging trends in fenestration design and performance hold promise for further improving window energy efficiency and occupant comfort. Some of these trends include:
6.1 Vacuum Insulated Glazing (VIG)
Vacuum insulated glazing (VIG) consists of two panes of glass separated by a vacuum space. The vacuum space eliminates conductive and convective heat transfer, resulting in extremely low U-factors. VIG offers significantly better insulation than conventional double- or triple-pane windows. However, VIG is currently more expensive than conventional glazing and requires special manufacturing techniques to maintain the vacuum seal. VIG is particularly advantageous in applications where space is limited, as it can achieve high insulation performance with a thinner profile than conventional glazing.
6.2 Chromogenic Materials
Chromogenic materials change their optical properties in response to an external stimulus, such as voltage, light, or temperature. Electrochromic, thermochromic, and photochromic windows are examples of dynamic glazing systems that utilize chromogenic materials. These systems offer dynamic control of the window’s energy performance, adapting to changing weather conditions and occupancy patterns. Research is ongoing to develop more efficient and cost-effective chromogenic materials and control systems.
6.3 Advanced Shading Strategies
Advanced shading strategies involve the use of sophisticated shading devices and control systems to optimize solar heat gain and daylighting. These strategies can include automated blinds, shades, and louvers that adjust their position based on weather conditions, occupancy patterns, and building orientation. Daylighting controls can dim or switch off electric lights when sufficient daylight is available, reducing energy consumption. Advanced shading strategies can significantly improve building energy performance and occupant comfort.
6.4 Integrated Fenestration Systems
Integrated fenestration systems combine multiple technologies into a single, integrated unit. These systems can include high-performance glazing, insulated frames, dynamic shading devices, and daylighting controls. Integrated fenestration systems offer optimized energy performance and occupant comfort, but they can be more expensive and complex to install than individual components.
6.5 Transparent Insulation Materials (TIMs)
Transparent Insulation Materials (TIMs) are materials that allow solar radiation to pass through while also providing thermal insulation. TIMs are typically used in passive solar heating applications. They can be incorporated into windows or walls to capture solar energy and reduce heat loss. Research is ongoing to develop more efficient and durable TIMs.
Many thanks to our sponsor Focus 360 Energy who helped us prepare this research report.
7. Conclusion
Fenestration systems, particularly windows, play a critical role in building energy performance. Older windows can be a major source of energy loss, leading to increased heating and cooling costs. The development and implementation of energy-efficient window technologies are essential for reducing building energy consumption and mitigating its environmental impact. Technologies such as multi-pane glazing, low-E coatings, gas fills, insulated frames, and dynamic glazing systems can significantly improve window energy performance. A comprehensive life-cycle cost analysis is necessary to evaluate the economic viability of window upgrades, considering regional climate variations and building characteristics. Emerging trends in fenestration design and performance, such as vacuum insulated glazing, chromogenic materials, and advanced shading strategies, hold promise for further improving window energy efficiency and occupant comfort. Policymakers, building design professionals, and homeowners should consider these advanced technologies when selecting or upgrading windows to minimize energy consumption and create more sustainable buildings.
Many thanks to our sponsor Focus 360 Energy who helped us prepare this research report.
References
- U.S. Department of Energy. (n.d.). Energy Saver: Windows. Retrieved from https://www.energy.gov/energysaver/windows
- Efficient Windows Collaborative. (n.d.). Understanding Windows. Retrieved from https://www.efficientwindows.org/
- ASHRAE Standard 90.1. (2019). Energy Standard for Buildings Except Low-Rise Residential Buildings. Atlanta, GA: ASHRAE.
- International Energy Conservation Code (IECC). (2021). Washington, D.C.: International Code Council.
- Griffith, B., et al. (2020). Achieving Zero Energy Buildings: Case Studies of Advanced Technologies and Design Approaches. Hoboken, NJ: John Wiley & Sons.
- Cellat, F., & Cetin, N. (2018). Dynamic windows: A review of recent developments and performance evaluation. Renewable and Sustainable Energy Reviews, 82, 2706-2718.
- Baek, N. C., & Park, J. (2021). A review of vacuum glazing technologies. Energy and Buildings, 231, 110596.
- Lee, E. S., DiBartolomeo, D., & Selkowitz, S. E. (2002). Impacts of low-e and high solar transmittance windows on energy performance and peak demand. Lawrence Berkeley National Laboratory.
- Arasteh, D., Apte, J., & Selkowitz, S. (1989). Window 3.1: a computer tool for analyzing window thermal performance. Lawrence Berkeley National Laboratory.
- Jelle, B. P., Hynd, A. W., & Gustavsen, A. (2012). Transparent insulation materials for building applications—a review. Energy and Buildings, 47, 161-171.
Fascinating report! Makes you wonder if we should all be living in giant thermos flasks. Considering the regional climate considerations, what about movable insulation, like window quilts? Could we bring back grandma’s craft project with a high-tech twist for optimal energy savings?
Thanks for the insightful comment! The idea of “high-tech” window quilts is intriguing. Integrating sensors and automated controls with traditional insulation could really optimize energy savings based on real-time conditions. This could be a great way to blend sustainability with personalized comfort. What materials do you think would work best?
Editor: FocusNews.Uk
Thank you to our Sponsor Focus 360 Energy
Considering the significant impact of window frames on overall heat transfer, what advancements are being made to enhance the thermal performance of frame materials beyond wood, vinyl, and fiberglass? Could aerogel or other novel insulators be integrated?
That’s a great question! The pursuit of enhanced frame materials is really exciting. Beyond the common materials, research is exploring composites with integrated aerogel or even vacuum-insulated panels directly within the frame. This could lead to significantly reduced thermal bridging. I wonder what the cost/benefit of the different materials are?
Editor: FocusNews.Uk
Thank you to our Sponsor Focus 360 Energy